17 Observing the ISM – IR, Optical & UV (Graded Reading)
Janice Hester
The interstellar medium (ISM) is composed almost entirely of hydrogen (about 75%) and helium. This gas is constantly heated by star formation, the light of the most massive stars, and the explosion of massive stars at the end of their “lives” (supernovae). It then cools again to become cold neutral hydrogen (mostly in the disk of the galaxy) and even colder and denser molecular clouds. These molecular clouds are then the site of new star formation. Imaging these many phases of the ISM requires observing over a wide range of wavelengths.
Optical, UV, and IR Imaging
For optical (visible), ultraviolet, and infrared imaging, astronomers use filters to capture images using different wavelengths of light. A telescope captures and focuses light across a wide range of wavelengths (e.g., by using mirrors that reflect IR, optical, and UV wavelengths). Filters allow a narrower range of wavelengths to reach a camera; for example, allowing only wavelengths close to yellow-green to pass through into the camera and form an image.
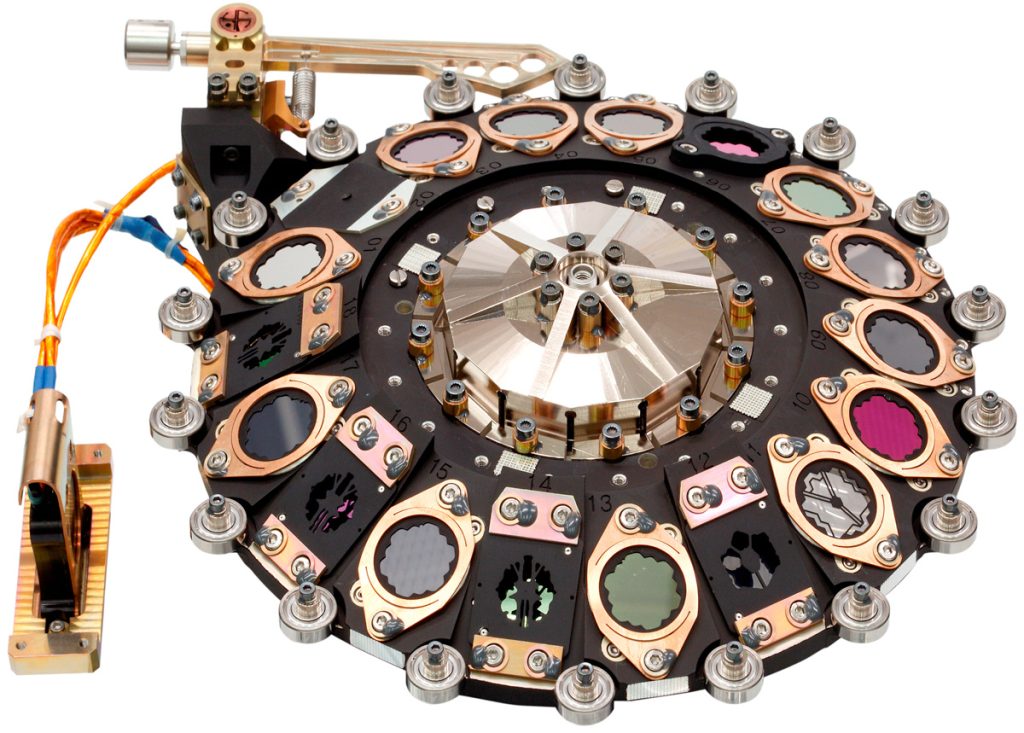
One example of using filters is creating color images. A typical color camera (like that in a smartphone) uses red, green, and blue filters to take three separate images. These three images are then colored and combined to create a full color image.
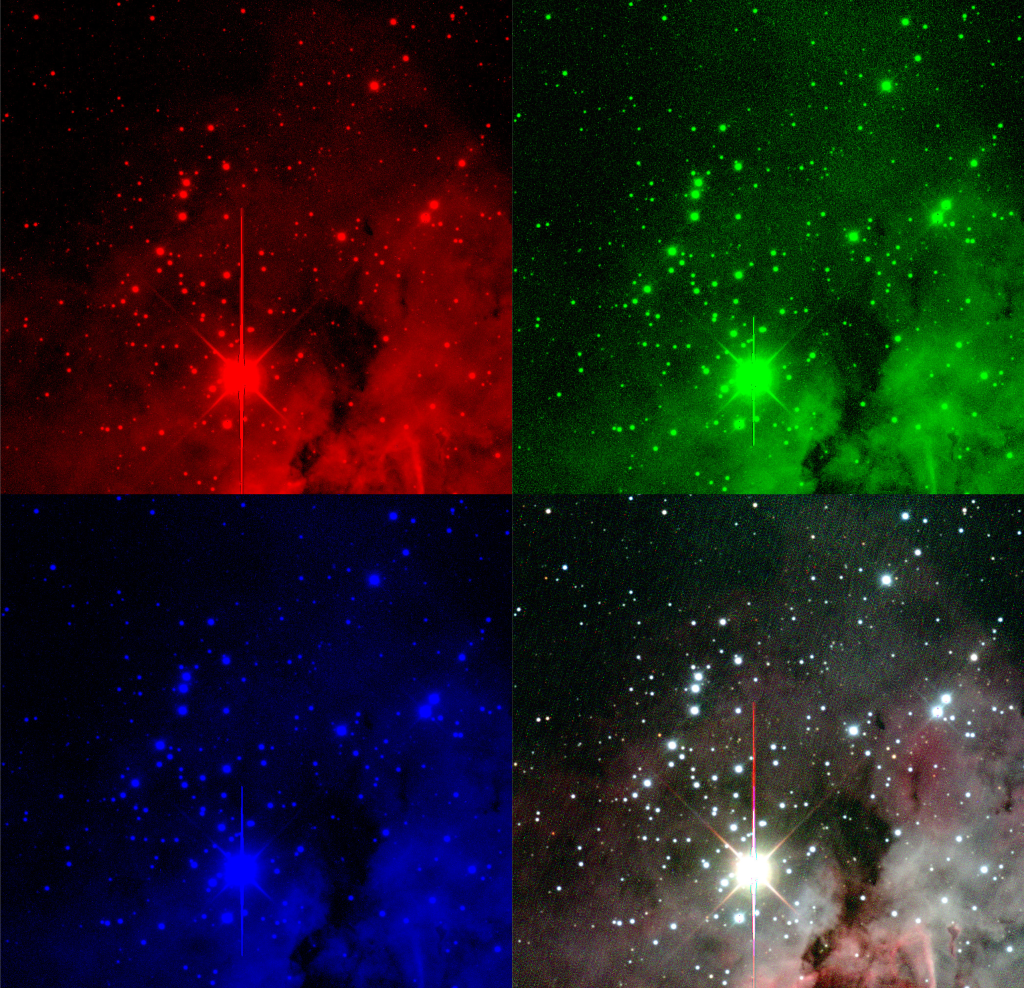
Astronomers use many different filters to capture specific wavelengths of UV, visible, and IR light. These filters can be roughly split into two types — broadband filters and narrowband filters. Broadband filters let in light across a broad range of wavelengths. For example, the Sloan r filter lets in light with wavelengths between about 550 and 700 nanometers (5500 and 7000 angstroms).
In contrast, narrowband filters let in light within a very narrow range of wavelengths. Narrowband filters are designed to capture specific emission lines from atoms (typically visible and UV emission lines) and molecules (typically IR emission lines). For example, an H-alpha filter lets in light with wavelengths close to the H-alpha emission line at 656.28 nm (the red hydrogen emission line). The goal when using a narrowband filter is to make an image that shows the location of the atoms or molecules that are emitting in the targeted emission line. Narrowband filters are often named for the element or ion responsible for the emission line that they are designed for.
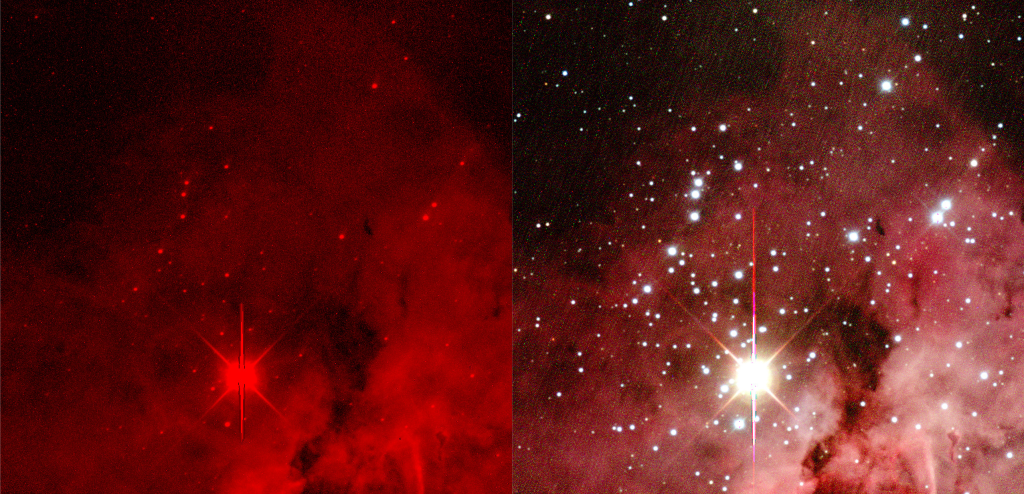
Broadband Imaging
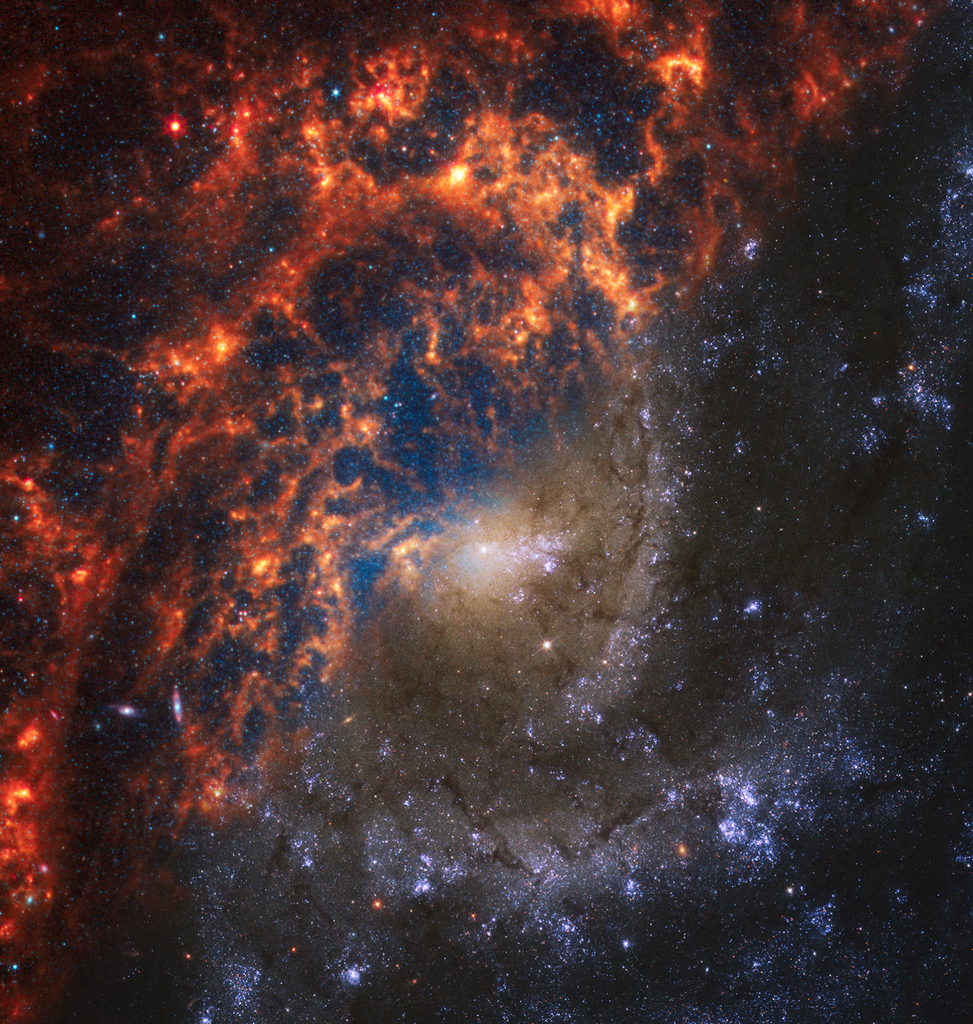
Broadband filters are good for capturing thermal emission, for example starlight and thermal emission from warm dust and hot gas both in our galaxy and in other galaxies. Starlight is emitted across wavelengths from the near-infrared (IR wavelengths that are just redder than red) through visible wavelengths and into the near UV (just bluer than blue). Different stellar types emit most strongly in different wavelengths, with O and B type stars shining brightly in the UV and stars closer to the Sun in mass shining most brightly in visible wavelengths.
Warm gas and dust — which is heated by star formation, hot young stars, and supernova explosions — glows in longer IR wavelengths. In the lower-right half of the image above, dust shows dark against the bright stars of the spiral galaxy. The stars shine in optical and UV light, which is absorbed by the dust, heating it. The warm dust glows in IR wavelengths, which is observed by the James Webb Space Telescope (JWST, upper-left). The hottest interstellar gas glows in the UV.
Filter System | Filters |
Johnson/Bessel | UBVRI |
Sloan/SDSS | ugriz |
Advanced Camera for Surveys (HST) – UV filters | f220w, f250w, f330s |
Near Infrared | IJHK |
Infrared Array Camera (Spitzer) | IRAC1-4 |
Narrowband Imaging
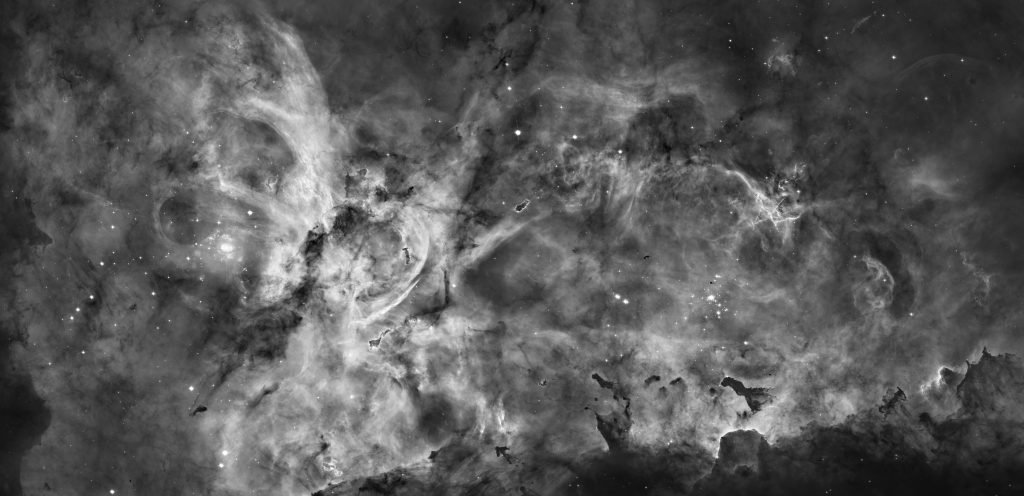
Image Source: https://hubblesite.org/contents/media/images/2007/16/2116-Image.html?page=5
Narrowband imaging is used to map the presence of the elements or molecules that are responsible for a particular emission line. In star forming regions, H-alpha imaging shows the presence of warm hydrogen that has been heated by the UV light from young massive stars. Ultraviolet photons have enough energy to ionize hydrogen; this is why these regions are called HII regions (HII is ionized hydrogen; HI is neutral hydrogen). The free electrons eventually recombine with a proton and cascade back down to the lowest energy level emitting photons along they way. The most common emitted photon is the red H-alpha photon, which is why star forming regions appear red.
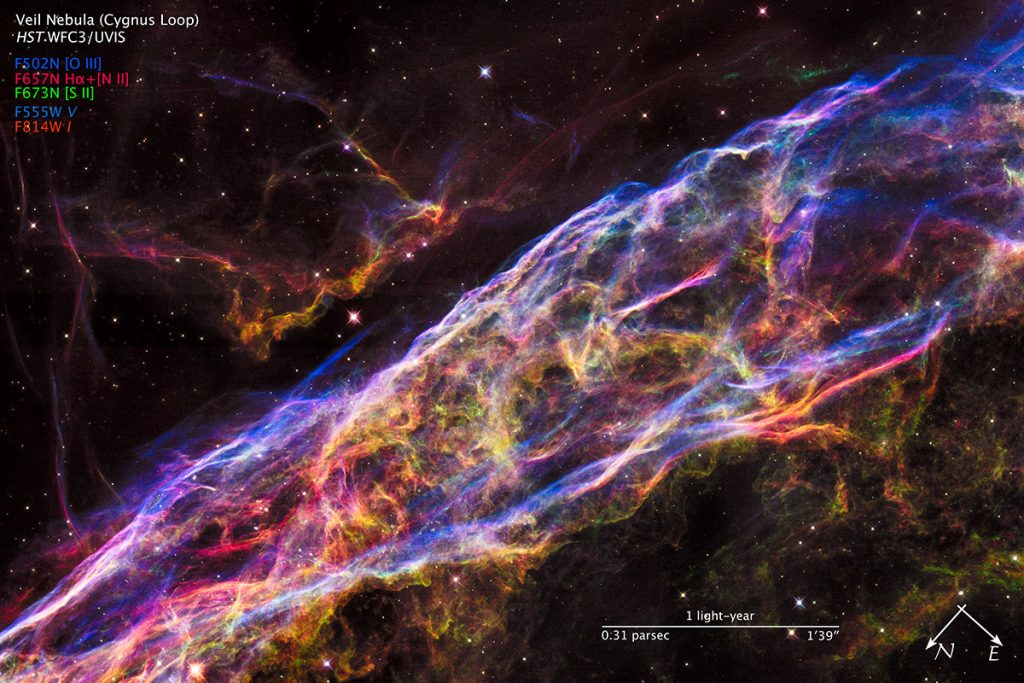
Supernova remnants – the gas left over from the explosion of a very massive star – are another popular target for narrow band imaging. When massive stars run out of hydrogen in their cores (because it has all been fused into helium) they start fusing heavier elements. Eventually, they run out of elements to fuse and explode as a supernova. The outer parts of the star are flung outwards while the inner part collapses to form a neutron star or black hole. More heavy elements are created during the supernova explosion. The material that is flung outward forms a supernova remnant. Supernova remnants are rich in elements other than hydrogen, including nitrogen (N), oxygen (O), and sulfur (S).