18 Observing the ISM – Radio & Microwave (Graded Reading)
Janice Hester
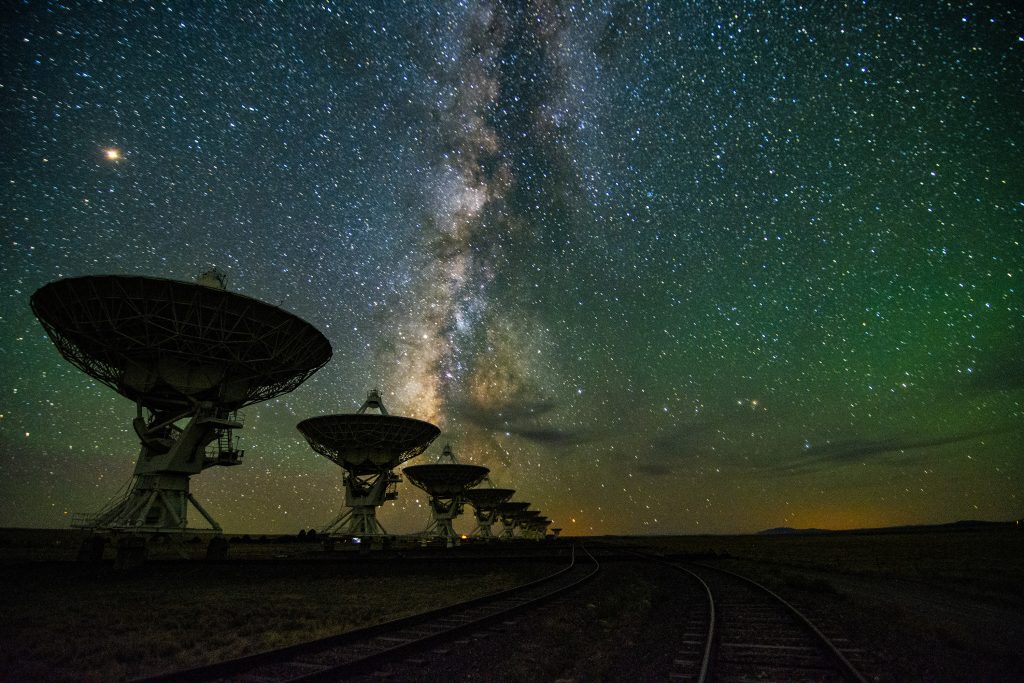
Neutral Hydrogen
Some of the most useful emission lines from the ISM occur at microwave and radio wavelengths. The hydrogen in HII regions (regions of ionized hydrogen) glows in H-alpha because the UV light from nearby young massive stars ionizes and excites the hydrogen atoms. Away from these hottest, most-massive stars hydrogen gas is cold enough to be in its ground (lowest energy) state and does not emit in any emission lines at UV, IR, or optical wavelengths.
Neutral hydrogen does, however, have an emission line in radio wavelengths. This low-energy emission line occurs because both the electron and the photon in the hydrogen atom have a spin, and these spins generate small magnetic fields. When the spin of the electron aligns with the spin of the proton, the magnetic fields also align, and the atom’s energy is slightly lower than when the two spin’s point in opposite directions.
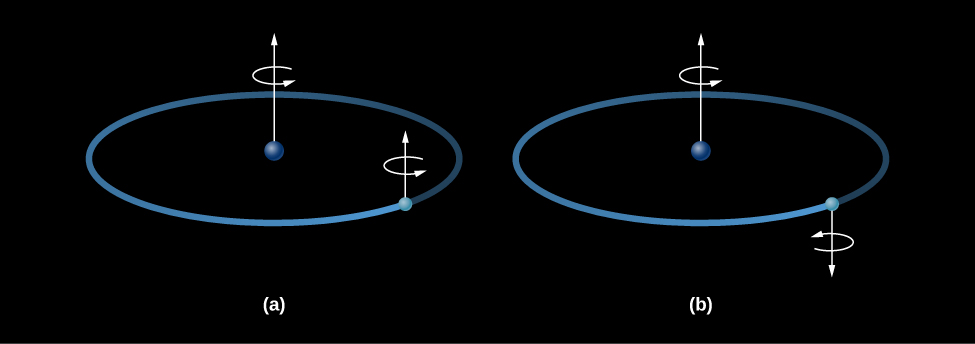
The direction of the electron’s spin is very unlikely to change, or flip, but when it does, a low energy photon is emitted (because the energy different between the two spins is small). This photon has a wavelength of 21 cm, which is in the radio portion of the electromagnetic spectrum. Fortunately, the galaxy is very large and contains a lot of neutral hydrogen, so even though this spin-flip transition is unlikely to happen in any individual atom, the Milky Way — and other spiral galaxies like it — are always glowing with 21-cm emission from cold, neutral hydrogen gas.
Rotation of the HI Disk
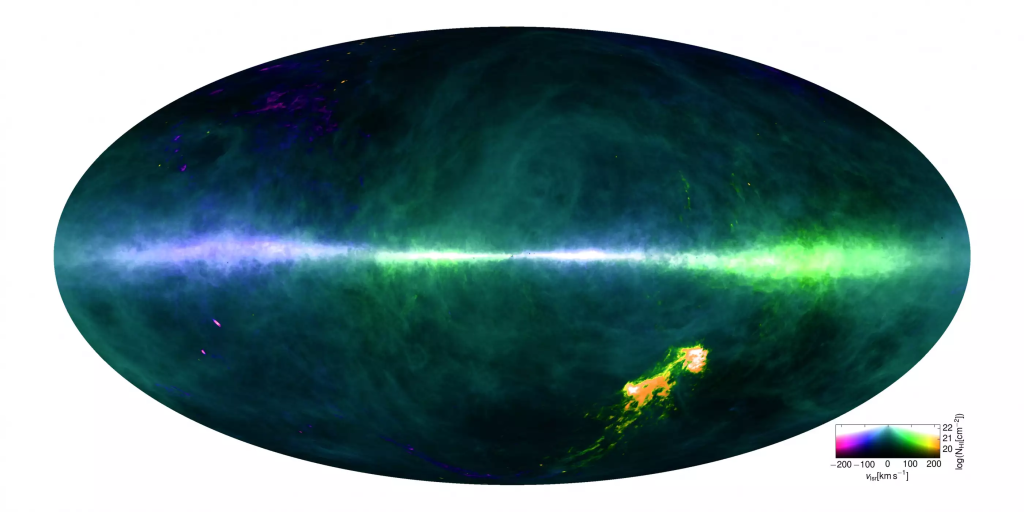
One advantage of observing in radio wavelengths is that, unlike optical filters, radio telescopes can be tuned to different frequencies while observing. This allows astronomers to detect the motion of the neutral hydrogen gas in the Milky Way and other galaxies. When hydrogen gas is moving towards us — the observers here on Earth — the observed emission lines are shifted to slightly shorter wavelengths (they are blueshifted). Emission lines from gas that is moving away from us are shifted to slightly longer wavelengths, or redshifted.
Using radio telescopes, astronomers have mapped the neutral hydrogen (HI) in the Milky Way and found that most of it is in a thin disk that rotates around the center of the galaxy. The pattern in the HI motions observed from our perspective reflects our perspective within the galaxy’s disk. In the all-sky image of HI emission (above), the central part of the map points towards the center of the galaxy and the edges point away from the galaxy’s center. The apparent (from our perspective) motion of the gas circling the galaxy’s center switches direction as we turn from looking towards the galaxy’s center (center of the image) to looking away from the galaxy’s center (edges of the image). This switch in apparent motion is seen in both the left and right halves of the HI map.
Molecular Clouds
In the densest and coldest part of the ISM, neutral hydrogen atoms bind together to form molecular hydrogen (H2). These molecular clouds also contain dark interstellar dust and molecules like carbon monoxide, ammonia, and various hydrocarbons. Inside the dense molecular clouds, these molecules are shielded from starlight, which would otherwise break them apart. It is also inside of molecular clouds that the gas can become dense enough to start collapsing under its own weight and form new stars.
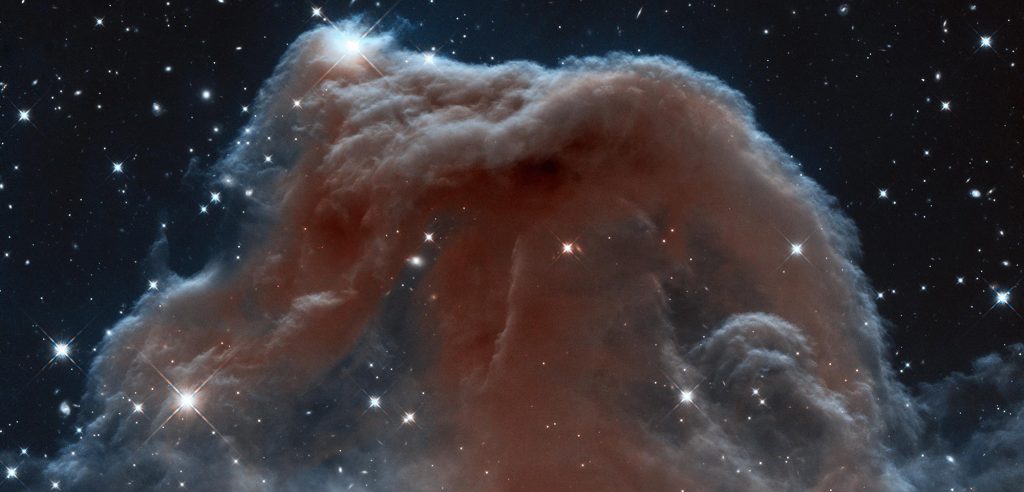
Nearby molecular clouds, like the Horsehead nebula shown above, can be observed when they block background stars or are warmed by nearby bright stars and glow in the infrared. More distant molecular clouds are difficult to observe because there are no easily observable emission lines that we can use to detect molecular hydrogen. Instead, molecular clouds are observed using emission lines from carbon monoxide (CO), one of the molecules found inside of molecular clouds.
Molecules have two kinds of emission lines. First, the electrons in the atoms that make up the molecule can change their orbital energy levels and emit photons at wavelengths that are characteristic of the individual atoms in the molecule. Second, the molecule itself has emission lines caused by the motions of the atoms within the molecule. Molecules can spin and flop and the bounds between atoms can bounce like springs. These motions store energy, which can be given up as a photon. Each molecule has distinct ways that it can move and therefore distinct photon energies and wavelengths that can be emitted. These molecular emission lines are typically in infrared, or longer, wavelengths.
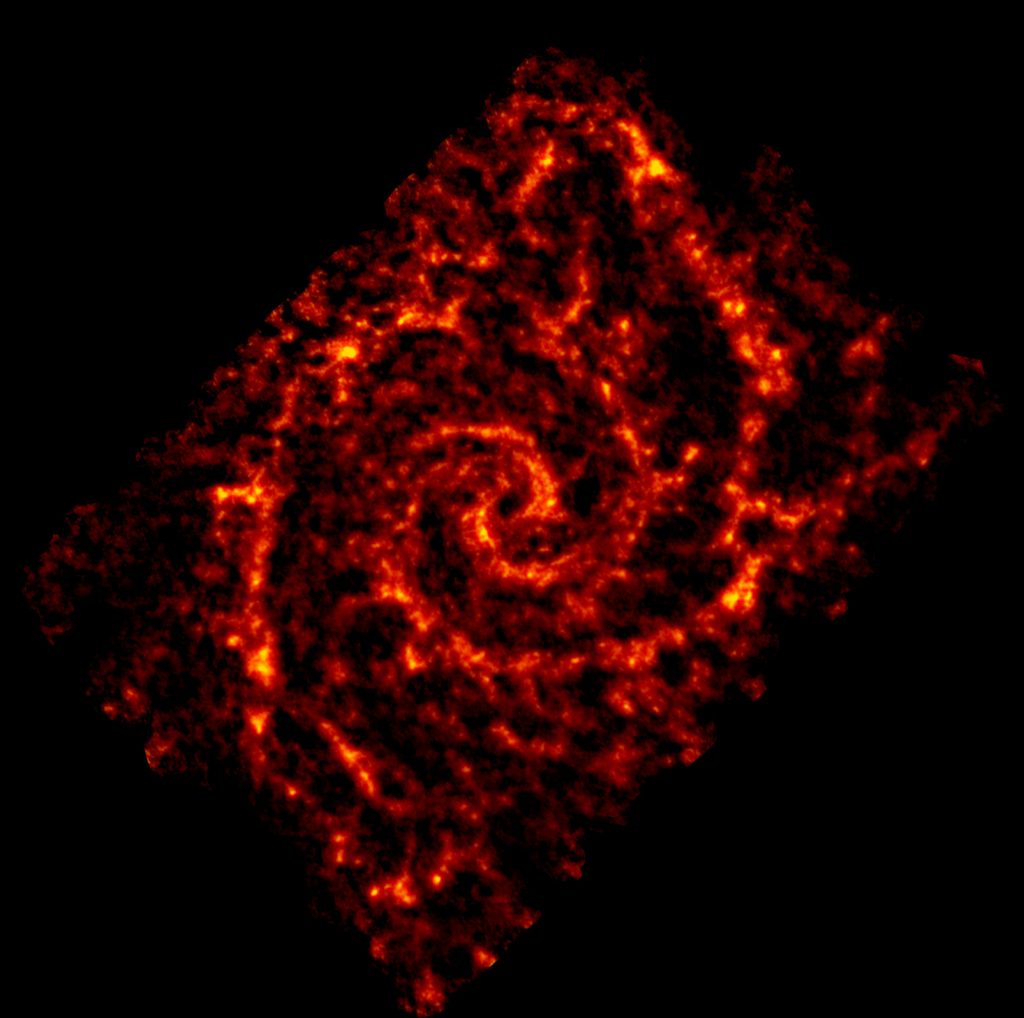
The carbon monoxide molecule has bright emission lines at millimeter wavelengths, which places them in the microwave part of the electromagnetic spectrum. Millimeter wavelength emission from carbon monoxide was first detected using a radio telescope at Kitt Peak Observatory in southern Arizona. Since then, the Atacama Large Millimeter/Submillimeter Array in the Atacama desert in Chile was built to make high resolution observations at millimeter wavelengths. The image above shows molecular clouds observed by ALMA in the spiral galaxy NGC 628. The molecular clouds trace the spiral arms of the galaxy. Observed in optical wavelengths these spiral arms glow blue with the light from the hot massive stars that are forming in these clouds.
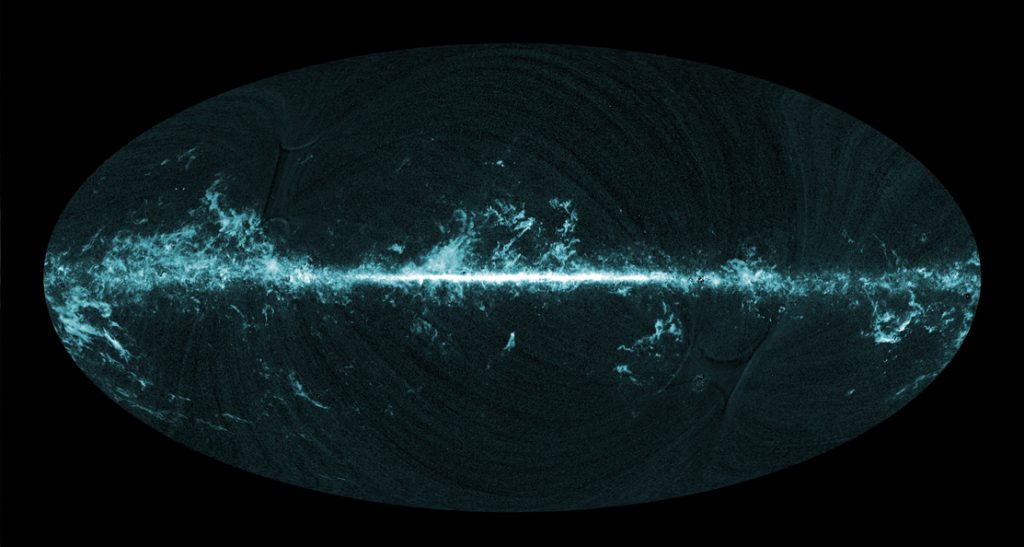
Observations of our own galaxy show that molecular clouds mostly form a very thin, cold disk within the disk of the Milky Way. Some molecular clouds, however, are found above and below the galactic plane. The all-sky CO map shown above was created with data from the Planck satellite, which was designed to observe the whole sky in microwave wavelengths in order to map the cosmic microwave background — the oldest light in the universe.
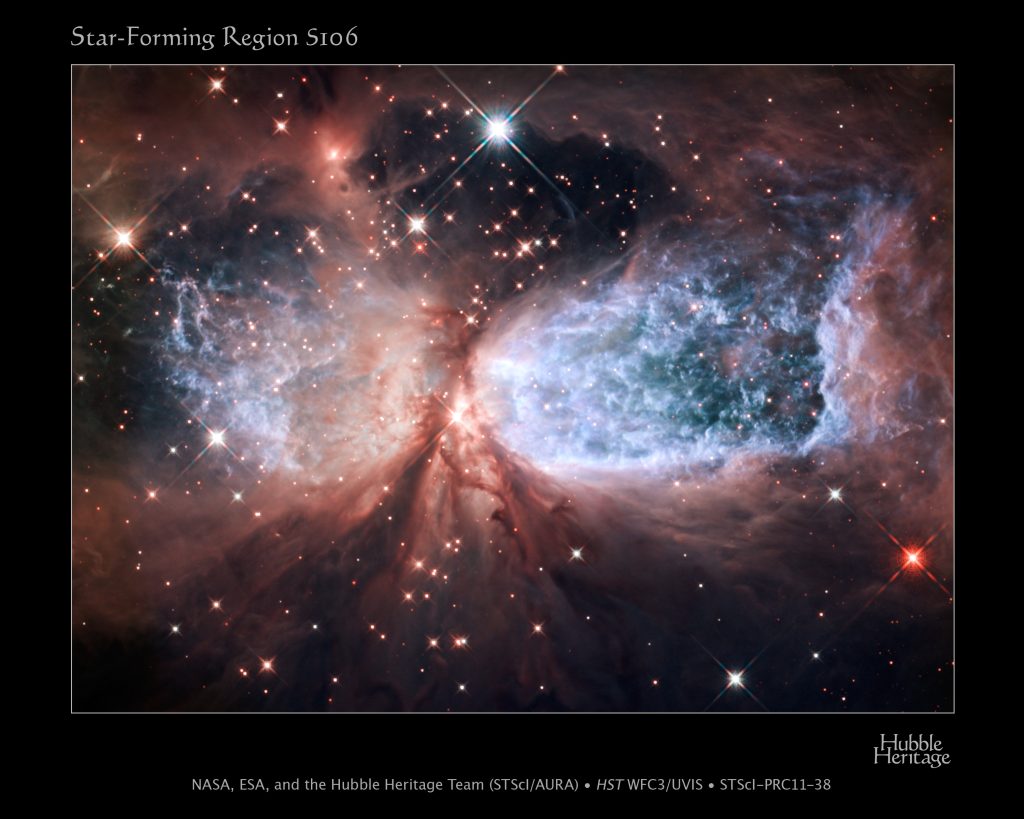
Molecular clouds are the site of star formation. Within a molecular cloud, stars of many masses are formed together. Stellar winds, UV light, and the eventual death of these stars – especially the most massive stars — disrupt the molecular cloud and halt star formation inside it. When hydrogen fusion begins inside of a star, a violent stellar wind blows away the surrounding gas and dust. These winds are particularly violent for massive stars (like the one at the center of the image above). Massive stars also emit a lot of UV light, which breaks the hydrogen molecules apart, ionizes hydrogen atoms, and heats the surrounding gas and dust — creating an HII region. Massive stars also quickly fuse through the hydrogen in their cores and explode as supernova, sometimes while less massive stars are still forming in the surrounding cloud.