15 Spectral Lines and Electron Orbitals (Graded Reading)
Janice Hester
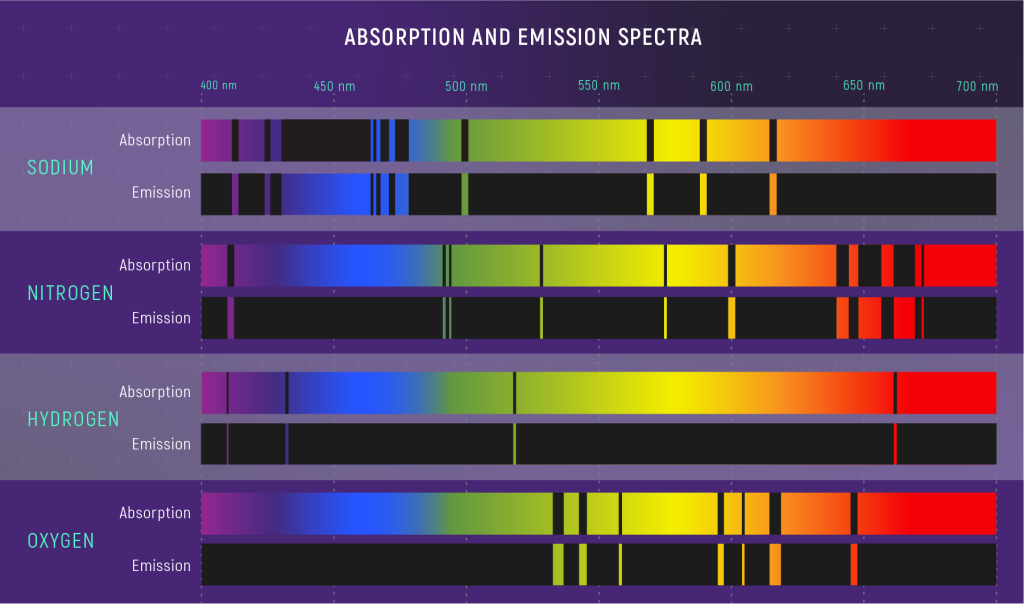
Emission and absorption lines are formed when atoms emit and absorb photons. When a photon is created, contributing to an emission line, the energy that the photon carries away must come from somewhere (remember that a photon is a packet of light energy). Similarly, when an atom absorbs a photon (contributing to an absorption line), the energy that the photon carried must go somewhere. For atomic lines (lines formed by atoms), the photon energy is transferred to and away from the binding energy of an electron in the atom.
Binding Energy
Within an atom, the negatively charged electrons are bound to the positively charged atomic nucleus. There is a force holding the electron around the nucleus, binding the electron to the atom. To remove an electron from an atom, you must figuratively “pull” it out of the atom. Energy is used to unbind the electron from the atom (this is called ionizing the atom). Conversely, when an electron becomes bound to an atom, energy is released.
Atomic binding energies are usually stated in units of electron volts (eV). One eV is [latex]1.602 \times 10^{-19}[/latex] joules of energy. A photon of light with a wavelength of 400 nm (violet light) has an energy of 3.10 eV. A photon of light with a wavelength of 700 nm (red light) has an energy of 1.77 eV. Ionizing (removing the electron from) the most tightly bound atom of hydrogen requires 13.6 eV of energy (a UV photon).
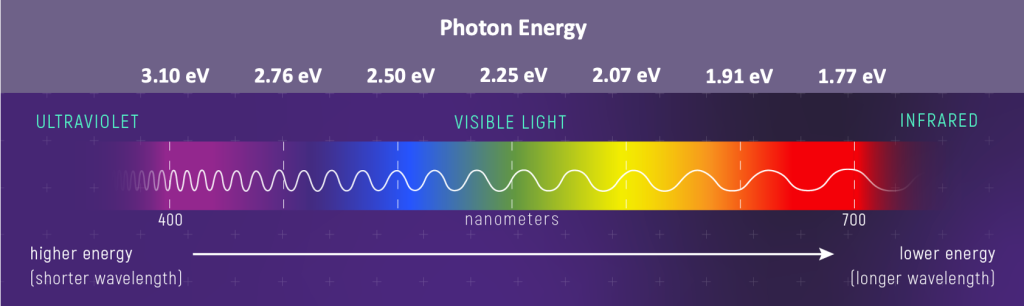
Atomic Energy Levels
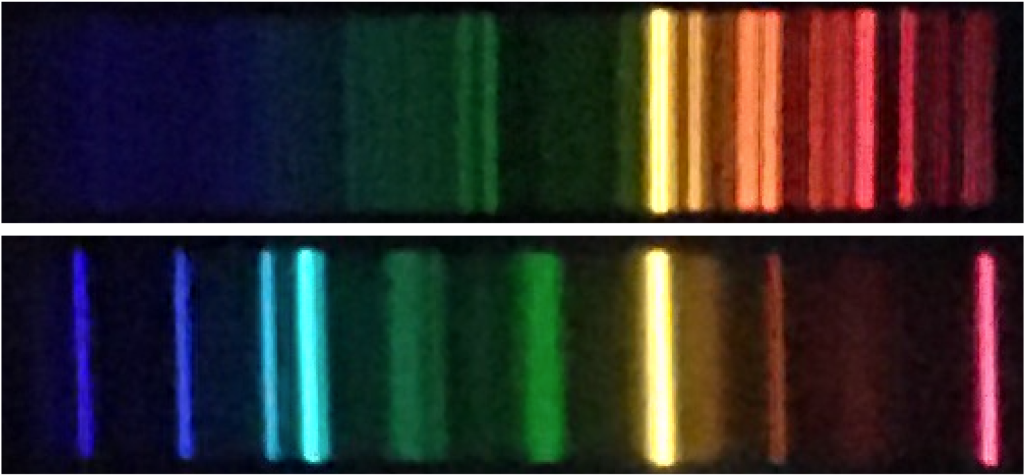
Electrons can be more or less tightly bound to an atom. When an atom absorbs a photon, one of the electrons inside it becomes less tightly bound to the atom. When an atom emits a photon, one of the electrons become more tightly bound.
The lines in atomic spectra show that atoms can only absorb and emit photons of specific energies. (Each single-color line in an atomic emission spectrum is made of many photons with the same energy.) This means that the energies (really energy deficits) binding electrons to atoms must also be specific energies. If electrons in an atom could have any binding energy, then they could absorb or emit photons with any energy. Instead, they have specific allowed binding energies, called energy levels.
Electrons in an atom can move between energy levels by emitting and absorbing photons. These photons have energies that equal the changes in energy as the electron moves between these energy levels.
Electron Orbitals
We know that electrons in an atom occupy specific energy levels because we can observe the lines in atomic spectra. We can also use the lines to measure the binding energies of the different energy levels. But this doesn’t explain why electrons in an atom have specific energy levels. The important concept for understanding electron energy levels is wave-particle duality, or the realization that at very small scales things don’t behave like a wave or like a particle, but a bit like each.
In our everyday experience, waves and particles (matter) are different. Mechanical waves move through matter, for example, across the surface of a pond or through air (sound waves). Waves can travel through each other or be layered on top of each other… multiple waves occupying the same place at one time. Matter doesn’t do this. Two waves directed towards each other will momentarily overlap as they pass through each other and continue on their way. Two softballs thrown at each other collide and bounce. Waves are oscillations (in matter for mechanical waves, or in the electric and magnetic fields, for light); they require constant change. A softball can rest on the ground indefinitely.
Light moves through space as waves in the electric and magnetic fields. But, light is created and destroyed as particles of light called photons. Similarly, electrons are particles. They can be created and destroyed, but they cannot be split in two. And also like light, electrons travel through space like a wave (not like a softball).
This is an important and surprising point; electrons travel through space like a wave. As strange as this idea is, we are very confident in it. The understanding that electrons travel like a wave underpins all of modern physics and many modern technologies. For example, the wave nature of electrons is the principle behind electron microscopy. In an electron microscope, a beam of electrons is focused by magnetic “lenses” and used to create an image of a specimen. Electrons have a very small wavelength, making them good for creating high resolution images of very small objects.
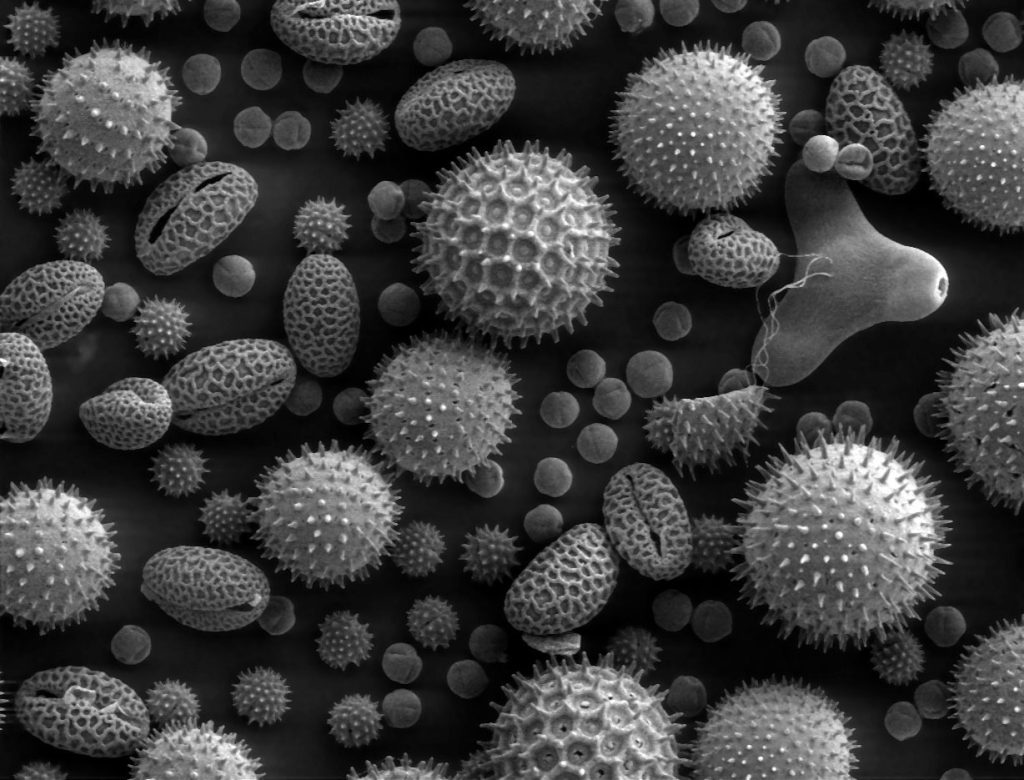
Because electrons move like a wave, not like a particle, they don’t orbit the nucleus in the same way that a planet orbits a star. Instead, within an atom, they exist as a complex wave pattern around the nucleus, called an orbital. (Electrons in orbitals don’t exist at a single point in space, but are spread out through space as a wave.)
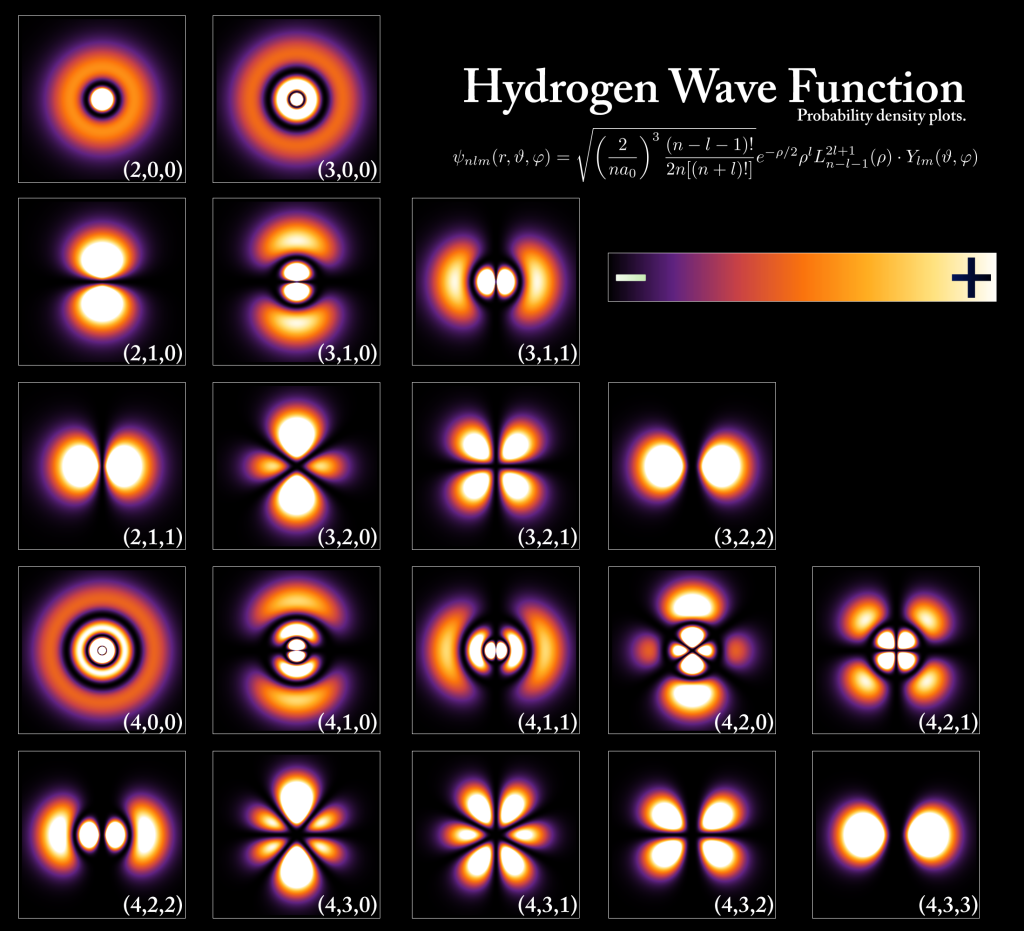
Orbitals are the only stable wave configurations within an atom, and each orbital has a specific binding energy, or energy level. An electron can remain in an orbital indefinitely, or can move between orbitals by absorbing and emitting energy. For example, within a hot gas, collisions between atoms cause electrons to move up to higher energy levels (a transfer of energy away from thermal motions in the gas). These electrons will eventually drop down to lower energy orbitals, emitting photons. This is the principle behind fluorescent lights.
Each element (and ion) has subtly different orbitals/energy levels. Increasing the number of protons in the nucleus tends to increase binding energies (making it harder to remove an electron), but electrons occupying more tightly bound orbitals make the least bound electrons in an atom easier to remove (lower binding energies). These differences lead to the unique spectra of each element.