10.4 Energy Resources: Uranium
Nuclear Energy
.
Nuclear energy is energy stored in the nucleus (core) of an atom. The forces that hold protons and neutrons together are incredibly strong [6]. When the nucleus of an atom splits, it releases energy in a process known as nuclear fission. During nuclear fission, a small atomic particle, a neutron, hits the uranium atom with enough energy to split its core, releasing heat, radiation. and more neutrons. These neutrons bombard other uranium atoms, and the process repeats itself. This is the chain reaction used by nuclear power plants to produce energy and transforms into electricity. Scientists and engineers have learned to split nuclei and control the release of energy to use it as a power source.
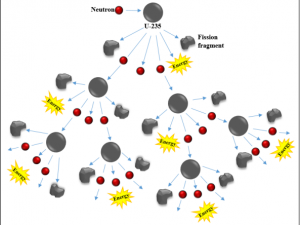

Uranium
Uranium is a chemical element, it has grey color and it was discovered in 1789 by a German physicist, M.H. Klaproth. Its chemical symbol is U and its atomical number is 92. It is a heavy metal, it is the heaviest natural element, 70% heavier than lead. It is also a naturally radioactive element that decays into daughter isotopes, releasing radiation energy. Uranium is a mixture of three different weights of atoms: three isotopes: 99.27 % uranium-238 (U238); the remainder consists of U-235 (0.72 %), and U-234 (0.006 %). U-235 is the preferred nuclear fuel because when its atoms are split (fissioned), they not only emit heat and high energy radiation but also enough neutrons to maintain a chain reaction and provide energy to power a 16 nuclear power plant. Uranium is found in rocks all over the world but is relatively rare and the supply is finite making it a nonrenewable energy source.

Uranium does not occur naturally in pure form. Instead, it usually occurs in combination with small amounts of other elements. Once mined, the U-235 must be extracted and processed before it can be used as a fuel in a nuclear power plant to generate electricity. The process begins with exploration for uranium and the development of mines to extract the discovered ore. Mining is either conventional (underground or open-pit) or unconventional, such as in-place solution mining or heap leaching, which uses liquid solvents to dissolve and extract the ore. Mined uranium ore typically yields one to four pounds of uranium concentrate per ton of uranium ore (0.05% to 0.20%) [6].
U Ore Deposits
The Colorado Plateau contains uranium ore deposits with different origins. Notably, the area surrounding Grand Canyon contains uranium accumulated in wreck rocks. This is how it works: The flow of groundwater dissolved parts of the Red Limestone Formation, creating underground sinkholes and caves. Sometimes, the dissolution and erosion move upward, generating hollow columns that at some point, collapse because of the weight of the overlying rock. The result is a “pipe” filled with breccia, thus the name for this deposit type is “breccia pipe” The fractured material acts as a trap for the uranium, which is transported by flowing groundwater. The breccia pipes could also contain copper, gold, silver, vanadium and other minerals. U ore deposits from different ages also occur in sedimentary rocks and are associated to water fluxes that transport the uranium.
U Processing
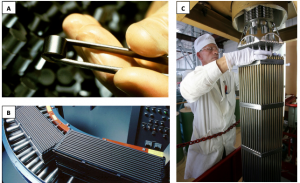
Uranium ore is refined into uranium concentrate in a process referred to as milling. The ore is crushed and ground into a fine powder, then it is reacted with chemicals to separate it from other minerals. The concentrated uranium product is typically a bright yellow or orange powder called yellowcake (U3O8). Milling operations produce tailings, which are an environmental liability. The yellowcake then undergoes conversion into uranium hexafluoride (UF6) gas. This step enables the atomic segregation of the three naturally occurring uranium isotopes into individual components. In the UF6 gas, the original concentrations of uranium isotopes are still unchanged. This gas is then sent to an enrichment plant where the isotope separation takes place and the concentration of U-235 is increased to about 4% to 5% (compared to 0.72% original concentration). The product, called enriched UF6, is sealed in canisters and allowed to cool and solidify before it is transported to a fuel assembly plant. The next step in the production of nuclear fuel takes place at fuel fabrication facilities. Here, the enriched UF6 gas is reacted to form a black uranium dioxide (UO2) powder. The powder is then compressed and formed into the shape of small ceramic fuel pellets. Each ceramic pellet produces roughly the same amount of energy as 150 gallons of oil. The pellets are stacked and sealed into long metal tubes that are about 1 centimeter in diameter to form fuel rods. Fuel rods are then bundled together to make up a fuel assembly. Depending on the reactor type, there are about 179 to 264 fuel rods in each fuel assembly. A typical reactor core holds 121 to 193 fuel assemblies [6].
Uses in Nuclear Energy
Nuclear power plants, such as Palo Verde nuclear station, in Arizona (Figure 10.15), use uranium, which is mined, processed, and then concentrated into fuel rods. According to the EIA, IN 2018 the Palo Verde generated a net summer capacity of 3,937 megawatts, making it the largest net generator of electricity. Coal, natural gas, nuclear power satisfied 88% of energy needs in Arizona in 2018. The numbers have changed after the closure of the Navajo Generating Station but the most recent data is not published.

Benefits of Nuclear Energy
Nuclear power plants generate electricity without emitting air pollutants like those emitted by fossil fuel-fired power plants. This means that financial costs related to chronic health problems caused by air pollutants such as particulate material, carbon monoxide, nitrogen oxides, and ozone among others are significantly reduced. Nuclear reactors do not produce carbon dioxide while operating, so nuclear energy does not contribute to the global warming problem. Another benefit of nuclear energy over fossil fuels, especially coal, is that uranium is more efficient, generating far more power per unit weight or volume than coal [6]. Nuclear Energy is clean, but “there’s no such thing as a free lunch” and the cost for it can be very high, especially for the communities where the uranium is found and processed.
The Drawbacks of Nuclear Energy
Radioactive Waste
The creation and disposal of radioactive wastes is the main environmental concern. Waste includes tailings from mining and from processing, spent (used) reactor fuel, dust, and other radioactive wastes. These materials remain dangerous to human health for thousands of years. Radioactive wastes are classified as low-level or high radioactivity levels. By volume, most of the waste related to the nuclear power industry has a relatively low-level of radioactivity. Uranium mill tailings contain the radioactive element radium Ra, which decays to produce radon Rn, a radioactive gas. Most uranium mill tailings are placed near the processing facility or mill where they come from. Miners covered uranium mill tailings with a barrier of material such as clay to prevent radon from escaping into the atmosphere, topped by a layer of soil, rocks, or other materials to prevent erosion of the sealing barrier [6]. However, these solutions are only temporary in the face of Earth’s dynamic processes. Currently, there is no long-term solution for storing this waste.
Other types of low-level radioactive waste are tools, protective clothing, wiping cloths, and other disposable items that get contaminated with small amounts of radioactive dust or particles at nuclear fuel processing facilities and power plants. These materials are subject to special regulations that govern their handling, storage, and disposal so they will not come in contact with the outside environment. High-level radioactive waste consists of spent nuclear reactor fuel (i.e., fuel that is no longer useful for producing electricity). The spent reactor fuel is solid small fuel pellets in long metal tubes called rods (Fig 10.14). Spent reactor fuel assemblies are initially stored in specially designed pools of water, where the water cools the fuel and acts as a radiation shield. Spent reactor fuel assemblies can also be stored in specially designed dry storage containers. An increasing number of reactor operators now store their older spent fuel in dry storage facilities using special outdoor concrete or steel containers with air cooling. There is currently no permanent disposal facility in the United States for high-level nuclear waste.
The processes for mining and refining uranium ore and making reactor fuel require large amounts of energy. Nuclear power plants have large amounts of metal and concrete, which also require large amounts of energy to manufacture. If fossil fuels are used for mining and refining uranium ore or in constructing the nuclear plant, then the emissions from burning those fuels could be associated with the electricity that nuclear power plants generate (Fig. 10.16).
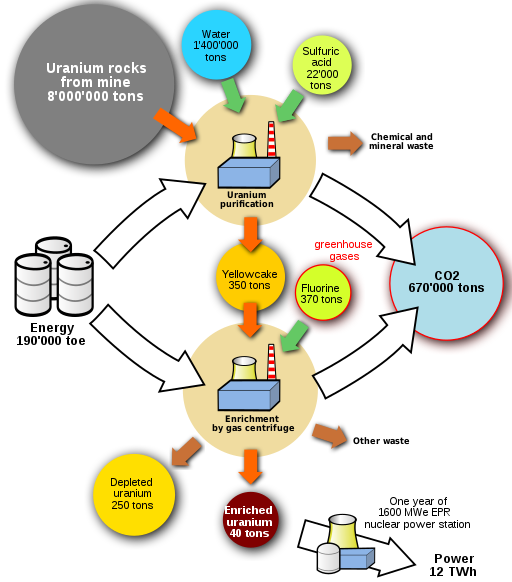
Impacts on health
U enters the body via ingestion, inhalation, and through the skin. Infrequent exposure to low levels of U is not considered problematic. In fact, the Colorado Plateau water contains natural low levels of U that are considered “safe”. However, mine workers and people living around processing facilities are continuously exposed to high levels of U and byproducts. Uranium contaminates soil, water, and air. Under this chronic exposure condition, U can accumulate in the kidneys, liver, muscles, bones, and the digestive track, and it will flow through the blood to reach the brain and interfere with other systems. U produces kidney damage, genetic damage, cancer, and developmental problems.
Nuclear Plant Failure
A nuclear meltdown, or uncontrolled nuclear reaction in a nuclear reactor, can potentially result in widespread contamination of air and water. Some serious nuclear and radiation accidents have occurred worldwide. The most severe accident was the Chernobyl accident of 1986 in the then Soviet Union (now Ukraine) which killed 31 people directly and sickened or caused cancer in thousands more. The Fukushima Daiichi nuclear disaster (2011) in Japan was caused by a 9.0 magnitude earthquake that shut down the power supply and a tsunami that flooded the plant’s emergency power supply. This resulted in the release of radioactivity although it did not directly result in any deaths at the time of the disaster. Another nuclear accident was the Three Mile Island accident (1979) in Pennsylvania, USA. This accident resulted in a near-disastrous core meltdown that was because of human error and mechanical failure but did not result in any deaths. While there are potentially devastating consequences to a nuclear meltdown, the likelihood of one occurring is extremely small. After every meltdown, including the 2011 Fukushima Daiichi disaster, new international regulations were put in place to prevent such an event from occurring again.
The development of nuclear power plants has been on hold for three decades. Accidents at Three Mile Island and Chernobyl, Ukraine verified people’s worst fears about the dangers of harnessing nuclear power.
Backyard Geology: Uranium in the Grand Canyon:
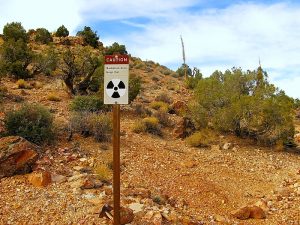
Nuclear energy does not contribute to climate change, but it comes at a high cost that is not evenly shared. Uranium mining and processing in Arizona have affected the lives and homes of the Native Americans. The Cold War-era provoked a uranium rush in the arms race between the US and the Soviet Union. To supply the enriched U, many mines opened in Northern Arizona, without regulations and without minimum safety measures to protect the workers. The war ended. Over 500 mines were abandoned but the contamination, environmental damage, and health problems did not leave and residents of the area cannot forget because they live in the contamination. Navajos have higher rates of cancer, larger than the rest of the population.
In the mid- 2000’s a spike in the U price brought back miners to the Colorado Plateau. Thousands filed claims around the Grand Canyon area. Because of the health and environmental risks, the Secretary of Interior at the time, Ken Salazar halted all claims in an area of one million acres of land for two years, to allow time for more research. In 2012, Salazar extended the moratorium 20 more years as a precaution.
However, in 2018, the Trump administration expressed interest in nuclear weapons and listed uranium as a critical mineral for National security, even though only non-fuel minerals should be listed. With this impulse, the mining sector pushed again to overturn the ban. However, preliminary results from the U.S. Geological Survey, show that the risk to the groundwater supply could be too high. An estimate of 30 million people would have their water source contaminated. The Red Wall aquifer, which underlies the Colorado Plateau and connects to the Colorado River, would be irreversibly contaminated. The Havasupai Tribe relies on this aquifer as their only source of water, the impact for them would be devastating. We also need more research to understand and measure the risk of Uranium mining. So far, the research has been largely underfunded.
The Grand Canyon is one of the Earth Wonders. People from all over the world come to admire it. The relentless erosion of the Colorado River has exposed its vast richness and wealth. We must not dig any further to find it. At least not until research has been properly funded and until we know exactly what to do when the aquifer gets contaminated, and until mining companies can be held accountable or choose to behave in an ethical way.
One of two or more species of the same chemical element, i.e., having the same number of protons in the nucleus, but differing from one another by having a different number of neturons
Valuable material in the Earth, typically used for metallic mineral resources.
A type of clastic sedimentary rock that consists of angular pebble or cobble-sized clasts that are cemented together with finer-grained sediments.