MICROBIAL METABOLISM
Americans eat 2 billion pounds of chocolate each year. The next time you bite into a delicious piece of chocolate consider this; microbial metabolism played a key role in how that chocolate became so tasty. Chocolate comes from cacao seeds found inside the pods of cacao trees. The pods of the cacao tree are split open and the seeds and the surrounding pulp are scooped out and placed on top of plantain or banana leaves. The seeds are then covered and left to ferment. Fermentation occurs as microorganisms including yeast and several kinds of bacteria grow on the fleshy, sugary pulp. Bathed in the fermenting pulp, the cacao seeds (which start off tasting bitter) begin to develop the flavors that we associate with chocolate. After fermentation the cacao seeds are dried, roasted, and then processed further by chocolate manufacturers before becoming one of our favorite desserts.
http://www.history.com/shows/modern-marvels/videos/how-chocolate-is-made
A steady supply of energy is necessary for microorganisms to perform the processes of life like protein synthesis, binary fission, flagellar motion, and endospore formation. Nutrient molecules have energy associated with the electrons that form bonds between their atoms. This energy is spread throughout the molecule and is difficult for microbes to utilize. Microbes liberate the energy from the bonds of the nutrient molecule and concentrate the energy into the bonds of ATP; ATP provides most of the energy for metabolism. In this review we will consider how microbes harvest the chemical energy stored in nutrient molecules (carbohydrates, proteins, and lipids) and use it to generate adenosine triphosphate (ATP).
How do disease-causing microbes acquire energy and nutrients at the expense of a patient’s health? How does grape juice turn into wine? How does yeast cause bread to rise? The answers to all these questions require an understanding of microbial metabolism. Metabolism comes from the Greek word metaballein-in Greek metaballein means change. Metabolism is defined as the collection of controlled biochemical reactions that take place within the microbe; all the chemical changes occurring in a microbe during its growth and development. Metabolism can be divided into two major classes of reactions: catabolism and anabolism.
Catabolism breaks down larger molecules into smaller molecules. Examples of catabolism are microbes breaking down glucose into carbon dioxide and water or breaking down lipids into glycerol and fatty acids. When catabolism occurs energy is released; microbes store some of this released energy in the bonds of ATP, though much of the energy released is lost as heat. Anabolism is the synthesis of larger molecules from smaller molecules. Examples of anabolism include the synthesis of proteins from amino acids or the synthesis of lipids from glycerol and fatty acids. Building things requires energy; the energy required for anabolism usually comes from ATP produced during catabolism.
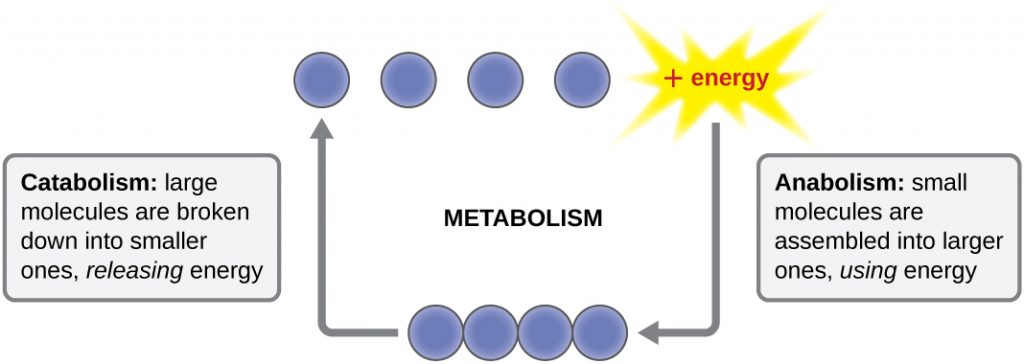
Image from openstax Microbiology
ATP
It has been estimated that a typical bacterium needs about three million ATP molecules per second to supply its energy needs. The ATP molecule is like a portable battery: it can move to any part of the microbe where energy is needed and supply the energy. ATP consists of adenine (a nitrogenous base) and ribose (a sugar), linked to three phosphate groups. ATP molecules are relatively unstable. Notice that the three phosphate groups all have negative charges on oxygen atoms. Like charges repel, so the phosphate groups in ATP, being tightly packed together, are very unstable. ATP is generally referred to as having high energy bonds. Actually, a better term is unstable bonds. Although the amount of energy in these bonds is not exceptionally large it can be released quickly and easily. ATP’s energy is released when an enzyme breaks the unstable bond connecting the third phosphate group to the remainder of the molecule.
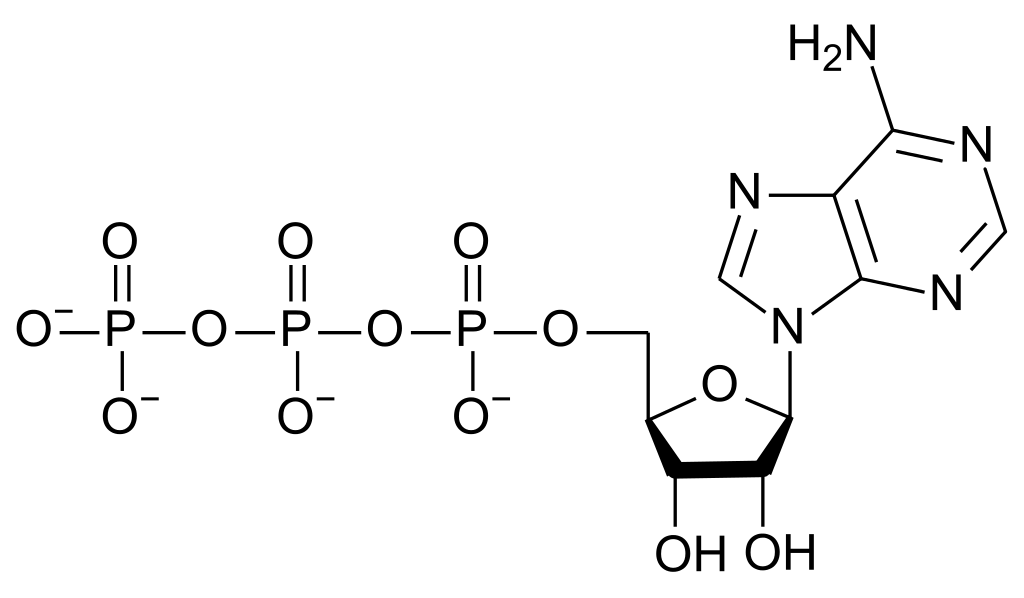
Carbohydrates, proteins, and lipids contain stored energy (in the electrons of their bonds) that can be extracted and transferred to ATP. With the help of enzymes, microbes extract the energy from nutrient molecules via a series of controlled reactions. Glucose is the most common carbohydrate used by microbes to produce ATP. Microbes can also use other carbohydrates, proteins, and lipids to produce ATP, we will discuss this later.
ATP can be produced from glucose while consuming oxygen in a process called cellular respiration or aerobic energy production. The equation for aerobic energy production is:
C6H12O6 + 6O2 ———->6CO2 +6H2O+ATP
Aerobic energy production has three steps: glycolysis, the Kreb’s cycle, and the electron transport chain.
glycolysis
Glycolysis takes place in the cytoplasm of both prokaryotic and eukaryotic cells. The term glycolysis is derived from glycol-referring to glucose and lysis-meaning to split. During glycolysis, glucose is converted into smaller molecules and eventually into the three carbon molecule pyruvic acid. Each of the steps of glycolysis is catalyzed by an enzyme. Overall glycolysis breaks down glucose into two pyruvic acid molecules with a net gain of two ATP and 2 NADH. NADH is an electron shuttle (carrier) holding electrons and their energy. NADH will shuttle the electrons and their energy to the electron transport chain. Glycolysis releases less than a quarter of the chemical energy in glucose; most of the energy remains stockpiled in the two molecules of pyruvic acid.
the bridge between glycolysis and the kreb’s cycle
Before pyruvic acid can enter the Kreb’s cycle it must first be converted to acetyl-coA. We will refer to this as the bridge between Glycolysis and the Kreb’s cycle. This conversion generates two CO2 molecules and 2 NADH molecules.
kreb’s cycle
Two acetyl-CoA molecules enter the Kreb’s cycle. In prokaryotes the Kreb’s cycle takes place in the cytoplasm. In eukaryotes the Kreb’s cycle takes place in the mitochondria, specifically the mitochondrial matrix. Each step of the Kreb’s cycle is catalyzed by an enzyme. The biochemical reactions in the Kreb’s cycle release the energy stored in the 2 acetyl CoA molecules. Overall the Kreb’s cycle produces 4 CO2 molecules, 6 NADH molecules, 2 FADH2 molecules, and 2 ATP molecules. FADH2 is another electron shuttle like NADH that will be used in the electron transport chain. In summary, glycolysis and the Kreb’s cycle have extracted as much energy as possible from glucose. The NADH and FADH2 produced during glycolysis and the Kreb’s cycle contain most of the energy originally stored in glucose.
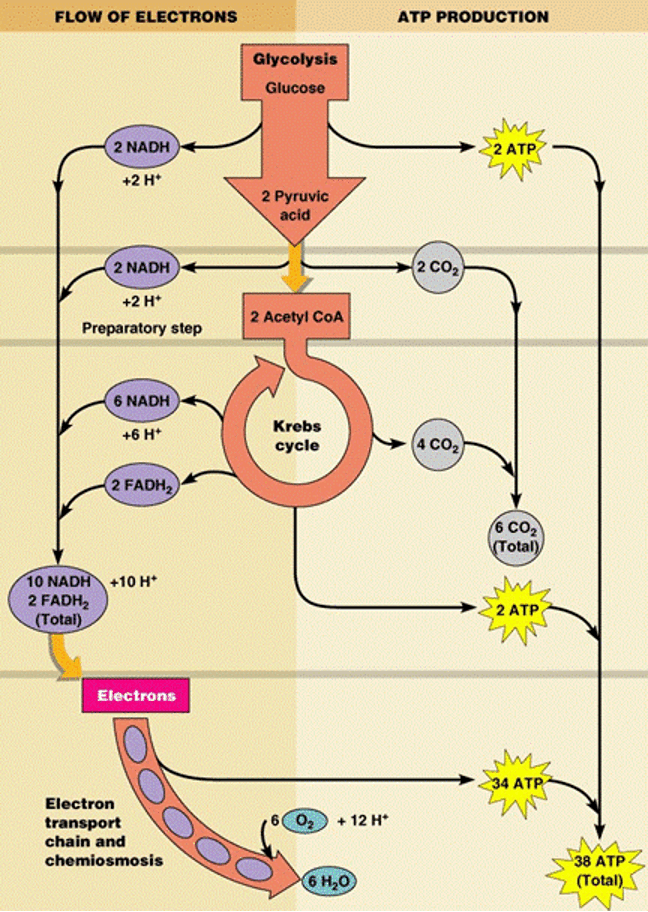
electron transport chain
The electron transport chain (ETC) is a series of proteins embedded in a membrane. In prokaryotes ETCs are embedded in the cell membrane. In eukaryotes ETCs are embedded in the inner mitochondrial membrane. Oxygen is the final member of the electron transport chain in aerobic energy production. The ETC is going to extract the energy from the electrons being held by NADH and FADH2. The energy that is extracted from the electron shuttles will then be stored in the bonds of ATP.
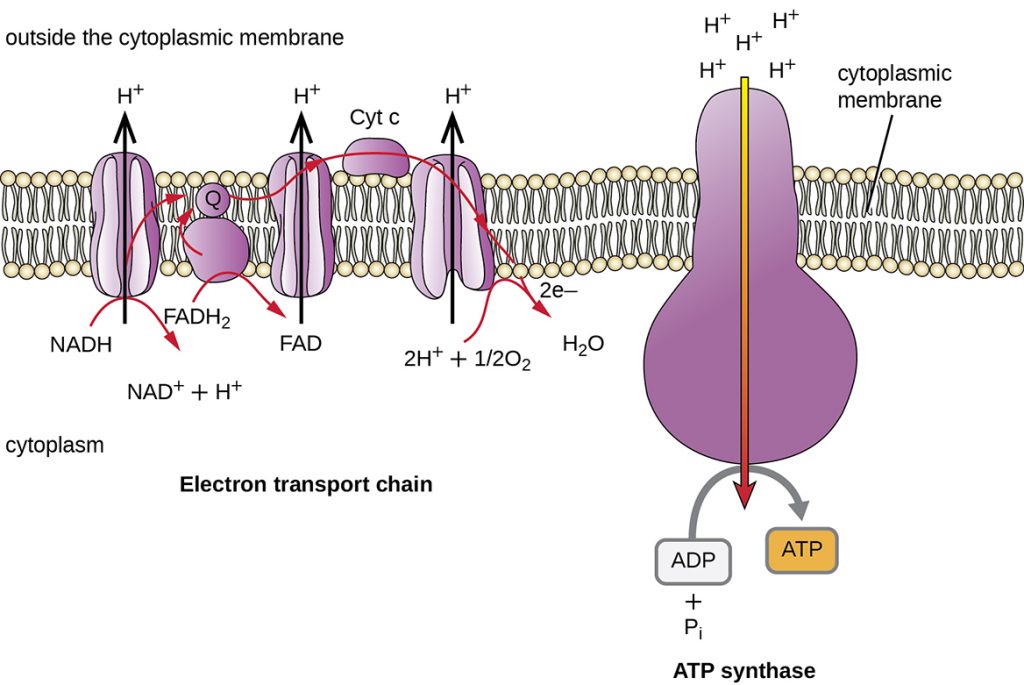
NADH drops its electrons off at the first member of the ETC. The electrons are passed down the members of the ETC. The electrons lose energy as they are passed down the ETC; the members of the ETC use that energy to pump hydrogen ions (H+) across the membrane. Oxygen is the final member of the ETC. The now non-energetic electrons and oxygen are converted to water. Pumping H+ across the membrane results in a high concentration of H+ on one side of the membrane and a low concentration of H+ on the other side of the membrane; a H+ concentration gradient has been formed. The H+ will flow back across the membrane via an ATP synthase. The force of the H+ concentration gradient will add the final phosphate group onto adenosine diphosphate to form ATP. The energy that was stored in electrons that NADH was carrying is now stored in the bonds of ATP.
FADH2 drops its electrons off a little further down the ETC. Because the electrons are dropped off further down the ETC a smaller H+ concentration gradient is built. For each NADH that drops its electrons off at the ETC 3 ATP are produced. For each FADH2 that drops its electrons off at the ETC 2 ATP are produced.
ATP accounting
Let’s do some accounting. In prokaryotes, aerobic energy production (glycolysis, Kreb’s cycle, and the ETC) produces 38 molecules of ATP from one molecule of glucose. In eukaryotes, aerobic energy production produces 36 molecules of ATP from one molecule of glucose. The yield of ATP is less in eukaryotes because they lose a little energy using the mitochondria. Let’s look at the overall equation for aerobic energy production again:
C6H12O6 + 6O2 ———->6CO2 +6H2O+ATP
Glucose feeds into glycolysis, oxygen is the final member of the ETC. Carbon dioxide is produced when pyruvic acid is converted to acetyl CoA during the Kreb’s cycle. The six carbons in glucose are now the six carbons in carbon dioxide. Water is produced during the ETC. The majority of the ATP produced in aerobic energy production comes from the ETC; a net of 2 ATP molecules are produced during glycolysis and 2 ATP molecules are produced during Kreb’s.
ATP Accounting Prokaryotes
STEP | ATP PRODUCED |
Glycolysis | 2 |
The Kreb’s Cycle | 2 |
The Electron Transport Chain | 34 |
Summary of Aerobic Energy Production
GLYCOLYSIS | THE BRIDGE | KREB’S CYCLE | ELECTRON TRANSPORT CHAIN | |
Enters | Glucose | 2 Pyruvic acid | 2 acetyl CoA | NADH, FADH2 |
Products | 2 Pyruvic acid, 2 ATP, 2 NADH | 2 acetyl CoA, 2 CO2, 2 NADH | 6 NADH, 2 FADH2, 4 CO2, 2 ATP | ATP, H2O |
Location in prokaryotes | Cytoplasm | Cytoplasm | Cytoplasm | Cell membrane |
Location in eukaryotes | Cytoplasm | Cytoplasm | Mitochondria (mitochondrial matrix) | Mitochondria (inner mitochondrial membrane) |
anaerobic energy production
Some prokaryotes live under anaerobic conditions. These microbes produce ATP via of anaerobic energy production. They use glycolysis, the Kreb’s cycle, and the ETC but oxygen is not the final member the ETC. These microbes use nitrate ions (NO-3) or sulfate ion (SO-4) as the final member of the ETC instead of oxygen. Because anaerobic energy production uses glycolysis, the Kreb’s cycle, and the ETC one molecule of glucose still produces 38 molecules of ATP.
fermentation
Fermentation involves only glycolysis. The pyruvic acid produced in glycolysis is transformed into other products like alcohol, lactic acid, and acetic acid. Since fermentation involves only glycolysis one molecule of glucose produces only 2 molecules of ATP. Fermentation is not lucrative in terms of ATP production and is not a long-term solution, cells use fermentation when electron acceptors like oxygen, nitrate and sulfate are not available.
PROCESS | Aerobic energy production | Anaerobic energy production | Fermentation |
ATP PRODUCED FROM ONE MOLECULE OF GLUCOSE | 38 prokaryotes, 36 eukaryotes | 38 ATP prokaryotes only | 2 ATP |
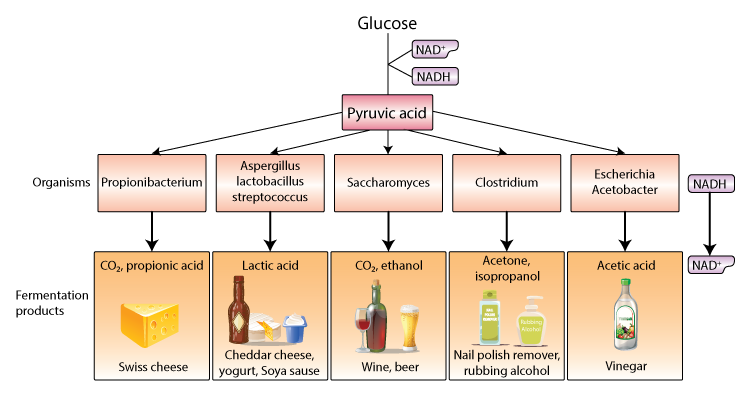
other fuels
We have been following the carbohydrate glucose through the ATP production process. Cells can use other nutrient molecules to produce ATP. Any carbohydrate can be used to produce ATP; it will feed somewhere into glycolysis. Lipids (or fats) can be used to produce ATP. Lipids are broken down into glycerol and fatty acids. Glycerol feeds into glycolysis, fatty acids feed into the Kreb’s cycle. Proteins also can be used to produce ATP. Proteins are broken down into amino acids. Amino acids feed into the Kreb’s cycle. Therefore, carbohydrates are the first choice for ATP production for cell because they produce the most molecules of ATP. Lipids are the second choice for ATP production and proteins are the third choice. Amino acids feed into the Kreb’s cycle so the cell loses the ATP and NADH it would have produced in glycolysis.
QuIZ TIME!
ADDITIONAL RESOURCES
https://openstax.org/books/biology-2e/pages/6-1-energy-and-metabolism