Chapter 5: Volcanoes
We may receive all the heat we need on the Earth’s surface from sunlight, but if you dig a hole just a few feet deep, you might notice that the surrounding soil is much cooler. This happens because much of the sediment is insulated from the sun’s energy. Nevertheless, if you had the ability to dig miles and miles into the crust, you would find that the temperature of the rock would start to significantly increase!

This increase in temperature with depth in the Earth’s crust is called the geothermal gradient. In general, we can expect temperatures to increase about 25°C for each kilometer of depth. This sounds like a significant increase in heat, but once we pass the 100 km mark, temperatures really take off!
As we learned in the second chapter, the Earth’s iron core is wickedly hot! Such heat powers plate tectonics, and when combined with other factors, it can lead to partial melting in the upper mantle. This melted, molten rock occasionally rises to the Earth’s surface and when it does, watch out!
A volcano will form above a chamber or plume of magma. There is a wide variety of volcanoes, and some are more explosive than others. Molten lava is far from the only hazard; volcanic eruptions can cause massive damage with ashfall, pyroclastic flows, toxic gases, and deadly landslides. There are even a few volcanoes considered so dangerous that they are termed “supervolcanoes”, and they have the potential to drastically impact society for generations.
Although volcanoes are hazardous, they are a continuous boon for the global economy. The precious minerals and useful rocks formed by volcanic processes have benefited civilization for thousands of years. In the modern economy, we use volcanism as a source of alternative energy and igneous rocks in trades ranging from construction to cosmetics.
Learning Objectives
- Describe the size, shape, and eruption style of volcanoes and how they form/
- Identify the major volcanic hazards and their effects on humans and property.
- Explain how scientists monitor volcanoes and where volcanoes can be found.
- Explain how volcanic processes benefit society.
The solidification of loose sediment materials as solid sedimentary rock through compacting pressures and cementation.
a pattern in which temperatures in the crust increase by about 25 degrees Celsius per kilometer (km) of depth.
a narrow piece of land that projects from a coastline into the sea
One of two or more species of the same chemical element, i.e., having the same number of protons in the nucleus, but differing from one another by having a different number of neturons
an area on the Earth's surface where lava, ash, and/or volatile gases erupt and eventually solidify into rock.
molten rock that can be found beneath the Earth's surface.
molten rock that has erupted at the Earth's surface due to volcanic processes.
It’s important to classify slope failures so that we can understand what causes them and learn how to mitigate their effects. The three criteria used to describe slope failures are:
- Material: Type of material that failed (typically either bedrock or unconsolidated sediment)
- Motion: How the material moved (fall, slide, or flow).
- Rate: Speed at which the material moved.
Three types of motion associated with slope failure are:
- Fall: Material drops through the air, vertically or nearly vertically.
- Slide: Material moves as a cohesive mass along a sloping surface.
- Flow: Material moves like a fluid.
Unfortunately it’s not normally that simple. Many slope failures involve two of these types of motion, some involve all three, and in many cases, it’s not easy to tell how the material moved. The types of slope failure that we’ll cover here are summarized in Table 11.1, though there are other types of mass wasting.
[Skip Table] | |||
Failure Type | Type of Material | Type of Motion | Rate of Motion |
---|---|---|---|
Rock fall | Bedrock | Fall | Extremely rapid |
Rock slide | Bedrock | Slide | Very slow to extremely rapid |
Rock avalanche | Bedrock (slides then breaks into smaller fragments) | Flow | Extremely rapid |
Creep or solifluction | Unconsolidated materials (rock fragments, soils) | Flow or slide | Extremely slow |
Slump | Unconsolidated sediments | Slide | Very slow to moderate |
Mudflow | Unconsolidated sediments (very small silt and clay) | Flow | Moderate to Extremely rapid |
Debris flow | Unconsolidated sediments (Sand, gravel, and larger fragments) | Flow | Rapid to Extremely rapid |
Rock Fall
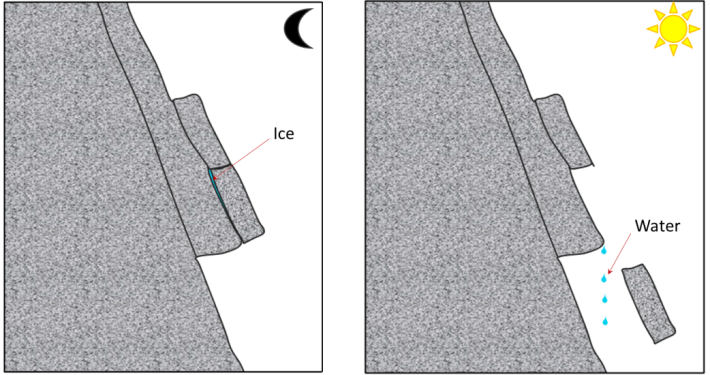
Rock fragments can break off relatively easily from steep bedrock slopes, most commonly due to frost-wedging in areas where there are many freeze-thaw cycles per year. When water freezes to form ice, its volume increases by about 8%, this causes fractures to enlarge. When the ice melts, more water can fill the larger crack, and refreeze. This process over time can cause large pieces of solid rock to fall (Fig 11.2.1). However, it can occur due to other triggers, particularly heavy rain, which adds weight or loosens material.
A rock fall in Verde Valley, Arizona, caused tons of solid material to fall to the valley below, destroying an RV but stopping short of hitting the house (Figure 11.2.2). Luckily no one was hurt in this rock fall, but living at the base of a cliff can be very dangerous and a fall is likely to happen again.

Rock Slide
A rock slide is the sliding motion of rock along a sloping surface. In most cases, the movement is parallel to a fracture, bedding, or metamorphic foliation plane, and it can range from very slow to moderately fast.
On June 23, 1925, a 38 million cubic meter rock slide occurred next to the Gros Ventre River (pronounced “grow vont”) near Jackson Hole, Wyoming. Large boulders dammed the Gros Ventre River and ran up the opposite side of the valley several hundred vertical feet. The dammed rivercreated Slide Lake, and two years later in 1927, lake levels rose high enough to destabilize the dam. The dam failed and caused a catastrophic flood that killed six people in the small downstream community of Kelly, Wyoming.

A combination of three factors caused the rock slide: 1) heavy rains and rapidly melting snow saturated the sandstone causing the underlying shale to lose its shear strength, 2) the Gros Ventre River cut through the sandstone creating an <span class="glossaryLink" style="border-bottom: 1px dotted #000000 !important;text-decoration: none !important;color: #000000 !important" aria-describedby="tt" data-cmtooltip="
">oversteepened slope, and 3) soil on top of the mountain became saturated with water due to poor drainage. The cross-section diagram shows how the parallel bedding planes between the sandstone and clay/limestone offered little friction against the slope surface as the river undercut the sandstone. Lastly, the rockslide may have been triggered by an earthquake. (3)
Rock Avalanche
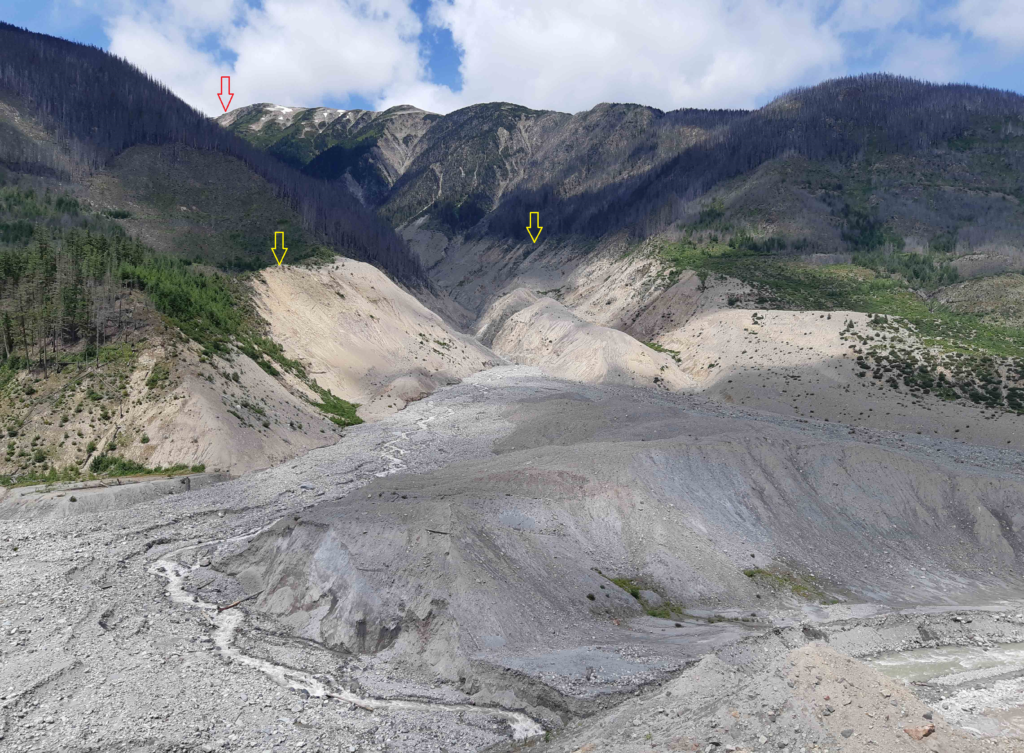
If a rock slides and then starts moving quickly (meters per second), the rock is likely to break into many small pieces, and at that point it turns into a rock avalanche in which the large and small fragments of rock move in a fluid manner supported by a cushion of air within and beneath the moving mass. The 2010 slide at Mount Meager (north of Vancouver, British Columbia, Canada) was a rock avalanche, and is the largest slope failure in Canada during historical times (Figure 11.2.5). Though no one was harmed during this event, there is a great potential for damage and loss of life from this type of mass wasting. (1)
Creep or Solifluction
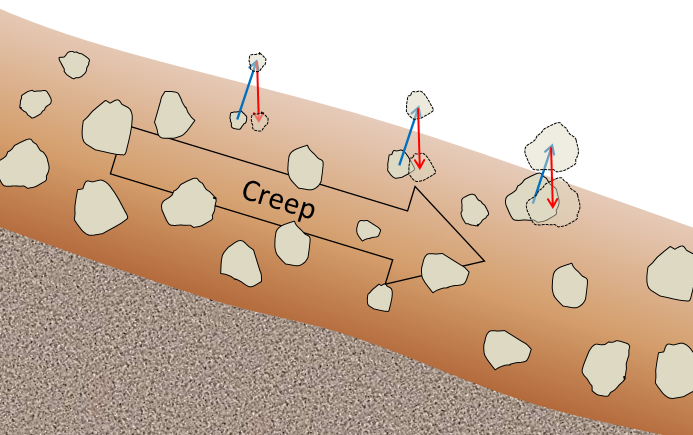
The very slow—millimeters per year to centimeters per year—movement of soil or other unconsolidated material on a slope is known as creep. Creep, which normally only affects the upper several centimeters of loose material, is typically a type of very slow flow, but in some cases, sliding may take place. Creep can be facilitated by freezing and thawing because, as shown in Figure 11.2.6, particles are lifted perpendicular to the surface by the growth of ice crystals within the soil, and then let down vertically by gravity when the ice melts. The same effect can be produced by frequent wetting and drying of the soil. In cold environments, solifluction is a more intense form of freeze-thaw-triggered creep. Creep is most noticeable on moderate-to-steep slopes where trees, fence posts, or grave markers are consistently leaning in a downhill direction. In the case of trees, they try to correct their lean by growing upright, and this leads to a curved lower trunk, shown on Figure 11.2.7.
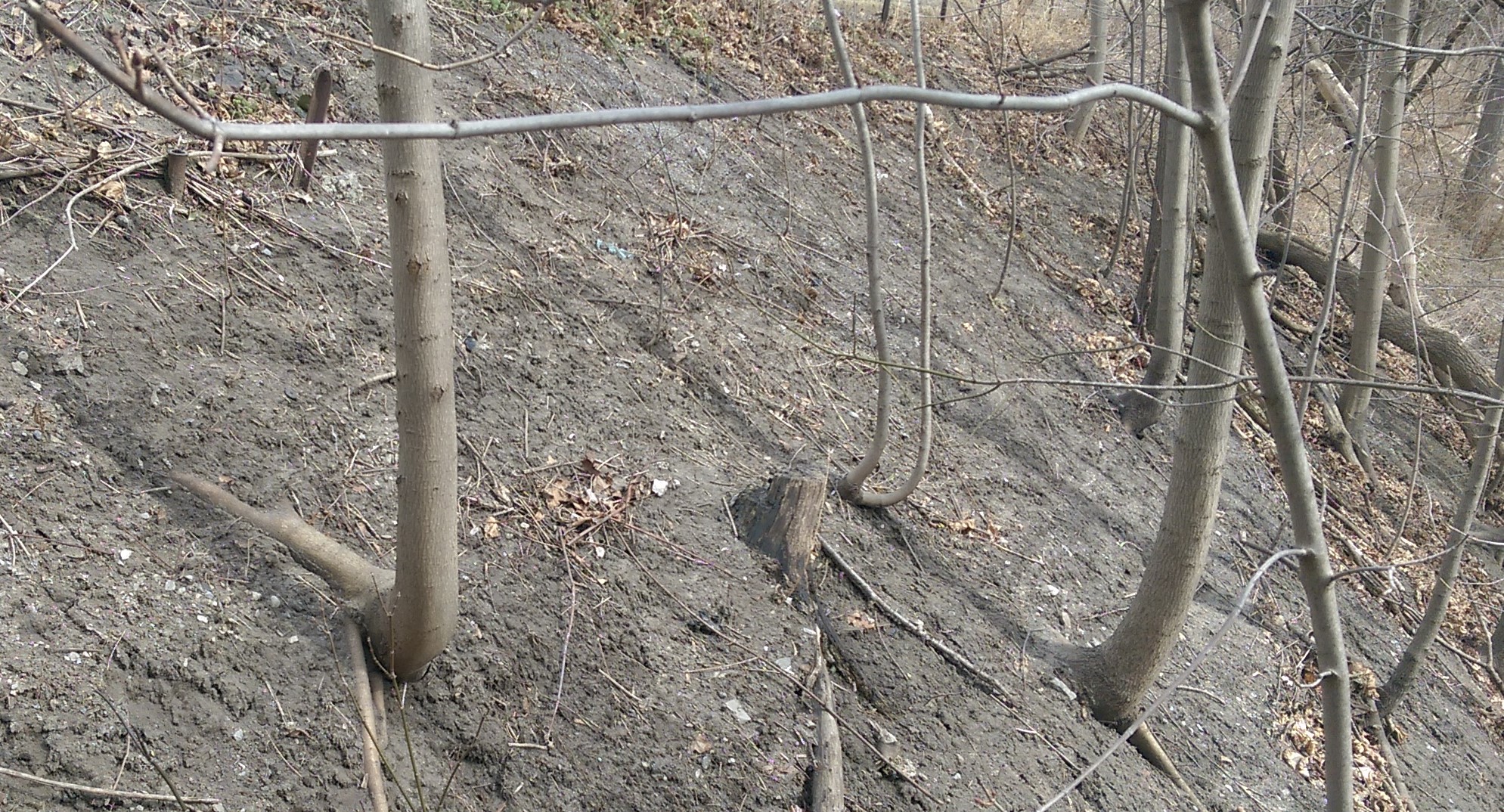
Slump
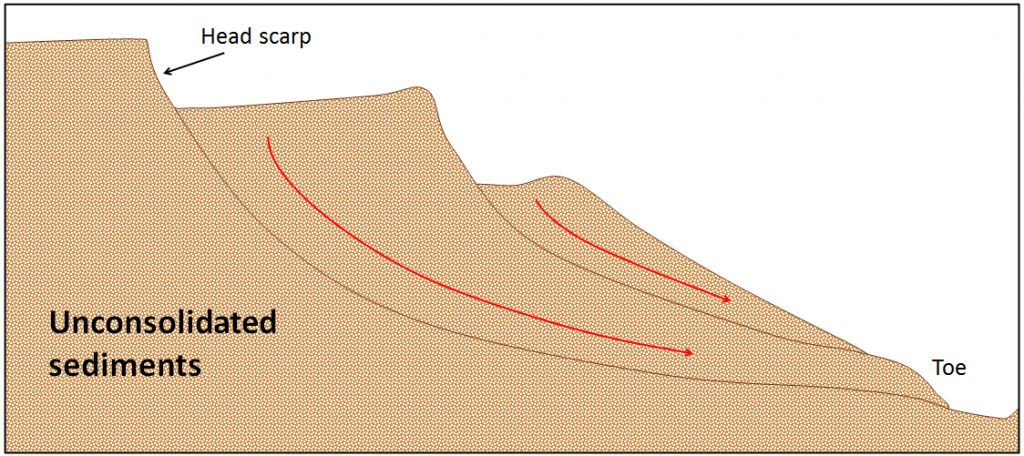
Slump is a type of slide (movement as a mass) that takes place within thick unconsolidated deposits (typically thicker than 10 meters). Slumps involve movement along one or more curved failure surfaces, with downward motion near the top and outward motion toward the bottom (Figure 11.2.8). They are typically caused by an excess of water within these materials on a steep slope.
An example of a slump in the Lethbridge area of Alberta is shown in Figure 11.2.9. This feature has likely been active for many decades, and moves a little more whenever there are heavy spring rains and significant snowmelt runoff. The toe of the slump is failing because it has been eroded by the small stream at the bottom.
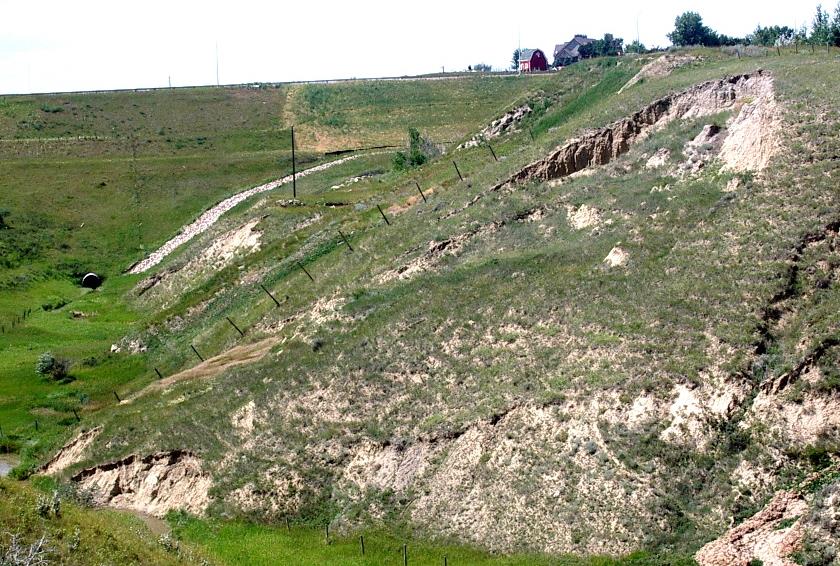
Mudflows and Debris Flows
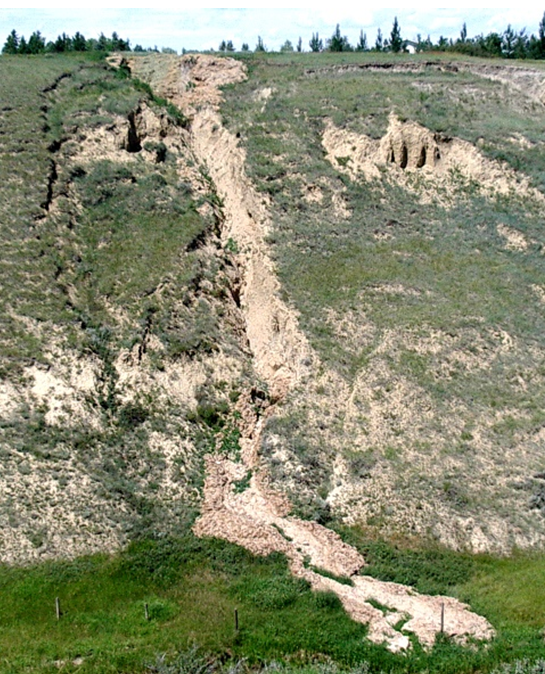
As you saw previously, when a mass of sediment becomes completely saturated with water, the mass loses strength, to the extent that the grains are pushed apart, and it will flow, even on a gentle slope. This can happen during rapid spring snowmelt or heavy rains, and is also relatively common during volcanic eruptions because of the rapid melting of snow and ice. (A mudflow or debris flow on a volcano or during a volcanic eruption is a lahar.) If the material involved is primarily sand-sized or smaller, it is known as a mudflow, such as the one shown in Figure 11.2.10.
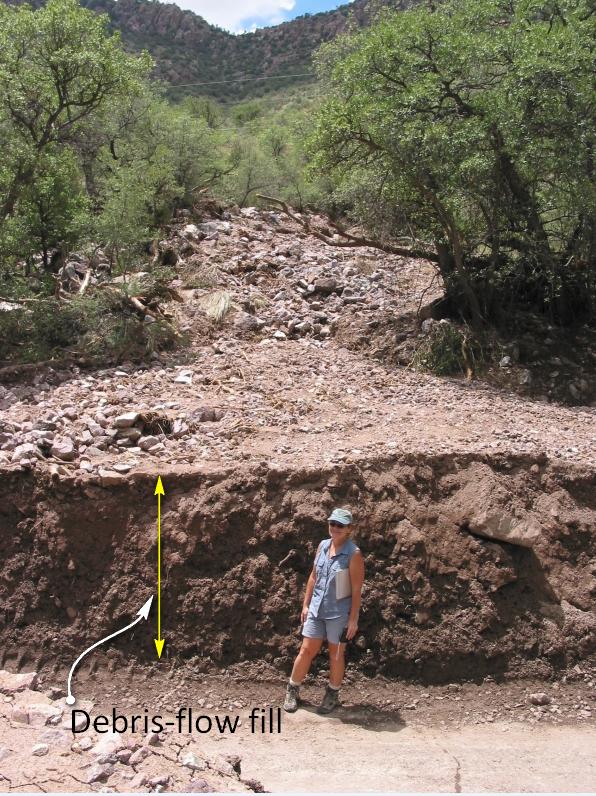
If the material involved is gravel sized or larger, it is known as a debris flow. Because it takes more gravitational energy to move larger particles, a debris flow typically forms in an area with steeper slopes and more water than does a mudflow. In many cases, a debris flow takes place within a steep stream channel, and is triggered by the collapse of bank material into the stream. This creates a temporary dam, and then a major flow of water and debris when the dam breaks. Large amounts of debris can be carried.
A region along Earth's lithosphere where at least two tectonic plates move apart from one another.
Volcanic Hazards
Volcanoes are responsible for a large number of deaths, but lava is not the only danger associated with these hazards. Mount Vesuvius (Naples, Italy) is infamous for its violent explosion thousands of years ago in 79 AD when a pyroclastic flow travelled over the Roman countryside and engulfed the cities of Pompeii and Herculaneum [1]. It was not until the 18th century that we uncovered the shocking remains of these towns beneath over 10 feet of ash and the casts of people preserved within it.
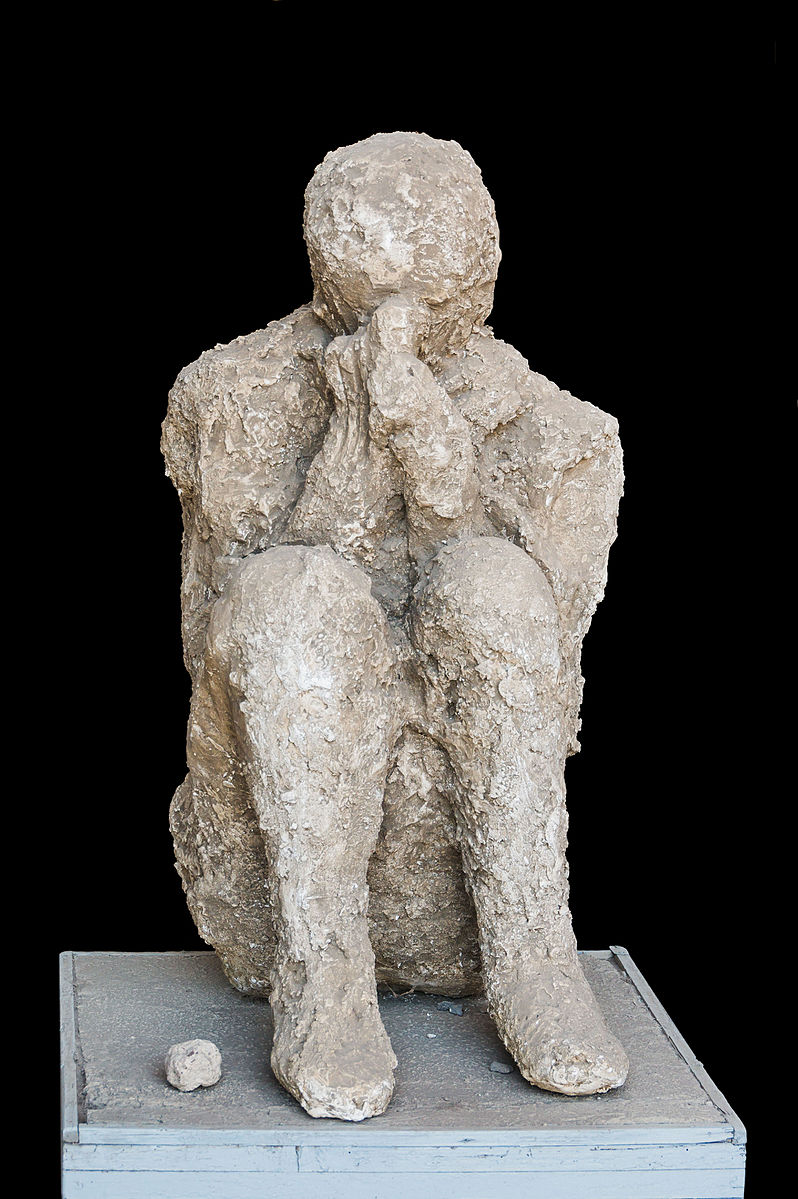
We know more about volcanic hazards over the past century because they have been better monitored and documented. We have seen enormously violent explosions, effusive lava, fast pyroclastic flows, ash, landslides, toxic gases, and more!
Pyroclastic Flows
The most dangerous type of volcanic hazard are pyroclastic flows. These flows are a mix of lava blocks, pumice, ash, and hot gases between 400 to 1,300°F! The turbulent cloud of ash and gas races down the steep flanks at high speeds at an average of 60 mph (much faster than people can run) into the valleys where farmlands grow and cities thrive [1].

Pyroclastic flows can often be expected of stratovolcanoes that contain felsic or intermediate magma. This magma is silica-rich and contains volatile gases that make it highly viscous. Therefore, these volcanoes often have very violent eruptions that are accompanied by a pyroclastic flow.
There are numerous examples of deadly pyroclastic flows. In 2014, the Mount Ontake pyroclastic flow in Japan killed 47 people. The flow was caused by magma heating groundwater into steam, which then rapidly ejected with ash and volcanic bombs. Some were killed by inhalation of toxic gases and hot ash, while volcanic bombs struck others [1]. In 1902, on the Caribbean Island Martinique, Mount Pelee erupted with a violent pyroclastic flow that destroyed the entire town of St. Pierre and killing 28,000 people in moments [3].
Lahars
https://www.youtube.com/watch?v=y9080YePWKY
A lahar is an Indonesian word for a mudflow that is a mixture of water, ash, rock fragments, and other debris that moves down the mountainside of a volcano (or other nearby mountains covered with freshly-erupted ash). They form from the rapid melting of snow or glaciers on volcanoes or sometimes in combination with a new eruption and heavy thunderstorm, as seen at Mt. Pinatubo.
Lahars move like a slurry of concrete, but they can move extremely fast at speeds up to 50 mph. Part of the reason they are so deadly is because they are slurry-like; they easily capture materials in their wake and they can travel very long distances like a flash flood [1].
During the 1980 Mount St. Helens eruption, lahars reached 17-miles (27 km) down the North Fork of the Toutle River. Another scenario played out when a lahar from the volcano Nevado del Ruiz Colombia, buried a town in 1985 and killed an estimated 25,000 people [1].

Tephra and Ash
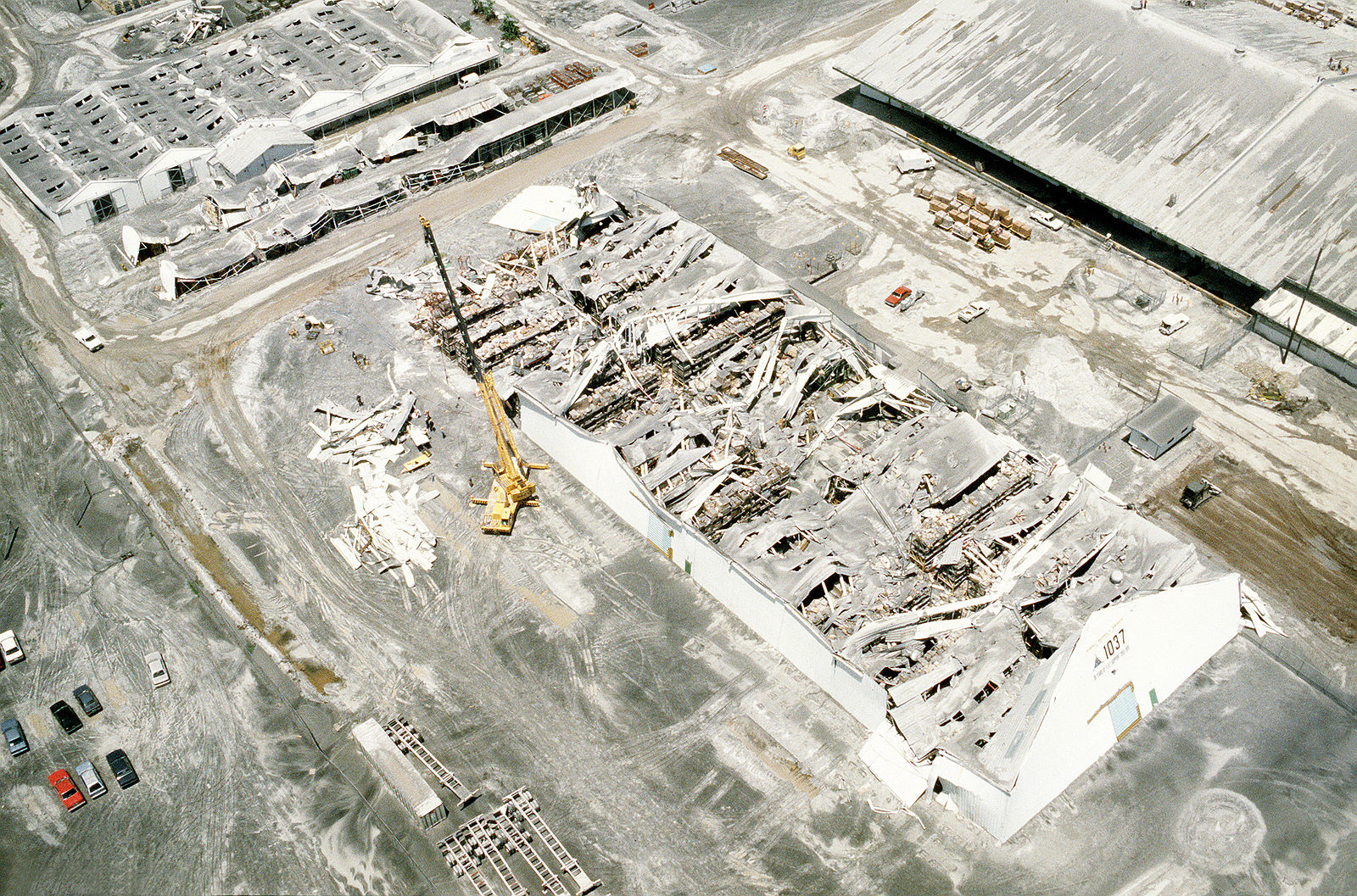
Volcanoes—mainly stratovolcanoes—eject substantial amounts of tephra and ash [1].
Tephra is heavier than ash, so it will fall closer to the volcano's crater and vent. Large masses of tephra sometimes erupt from volcanoes and can pose deadly hazards to anyone nearby. These are called volcanic bombs [1].

Ash is much finer, but it should not be underestimated. It can be carried much longer distances away from the volcano, and that can cause more widespread issues in nearby towns and cities. A build-up of ash can collapse the roof of a building or home, and the microscopic minerals within ash will cause respiratory illnesses such as silicosis. Inhaling ash is extremely hazardous because much of it contains microscopic volcanic glass particles [4]. Imagine inhaling tiny shards of glass!
Ash will also interfere with transportation services farther away from an eruption. For example, the 2010 Eyjafjallajökull volcanic eruption in Iceland created a gigantic ash cloud that caused a significant air travel disruption in northern Europe. No one was hurt, but the cost to the world economy was estimated to be billions of dollars [3].
Volcanic Gases
Magma contains dissolved volatile gases. As magma rises toward the surface, the pressure that keeps it in

the magma chamber will start to decrease, which allows those gases to escape. Think of this process like twisting the cap of a soda bottle; the first thing to rise and escape in that initial hissing noise is gas [1]!
The types of gases that are commonly released from volcanoes include greenhouse gases such as carbon dioxide (CO2), sulfur dioxide (SO2), hydrogen sulfide (H2S), methane (CH4), and water vapor (H2O). There are also toxic and acidic gases present at volcanoes such as HF, HBr, and HCl. After gigantic volcanic explosions, some volcanic gases such as sulfur dioxide will become sulfate aerosols in the atmosphere. These aerosol particles block sunlight coming toward Earth's surface and cause the planet to become cooler [5].
The volcanic gases can be both toxic and suffocating, and these gases sometimes are released from a volcano without an accompanying eruption. Gases were released from the Oku Volcanic Plain in Lake Nyos, Cameroon, and the carbon dioxide suffocated almost 2,000 people in 1986 [1].
Image by USGS (Public Domain).