11.1 Factors That Control Slope Stability
Mass wasting happens because tectonic processes have created uplift. Erosion, driven by gravity, is the inevitable response to that uplift, and various types of erosion, including mass wasting, have created slopes in the uplifted regions. Slope stability is ultimately determined by two factors: the angle of the slope and the strength of the materials on it. (1)
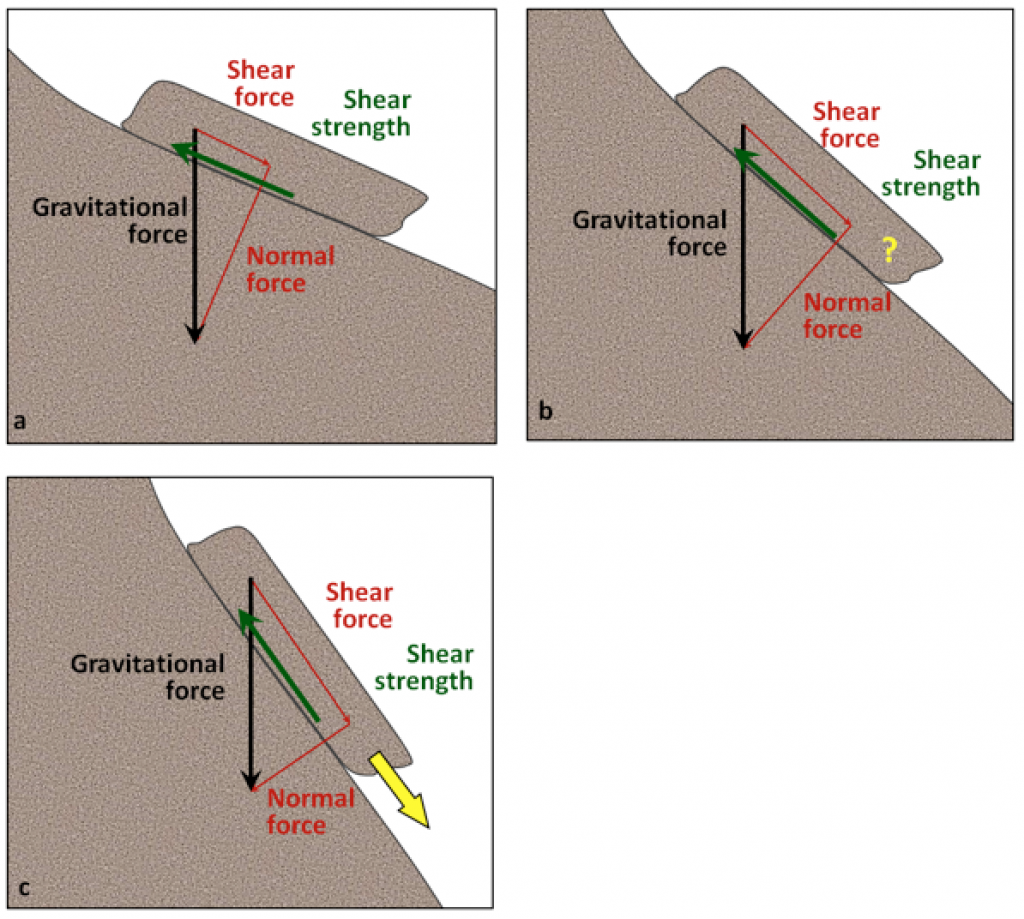
Figure 11.1.1 shows a block of rock situated on a rock slope. The block is being pulled toward Earth’s center (vertically down) by gravity. We can split the vertical gravitational force into two components relative to the slope: one pushing the block down the slope (the shear force), and the other pushing into the slope (normal force). The shear force, which wants to push the block down the slope, has to overcome the strength of the connection between the block and the slope, which may be quite weak if the block has split away from the main body of rock, or may be very strong if the block is still a part of the rock. This is the shear strength, and in Figure 11.1.1a, it is greater than the shear force, so the block should not move. In Figure 11.1.1b the slope is steeper and the shear force is approximately equal to the shear strength. The block may or may not move under these circumstances. In Figure 11.1.1c, the slope is steeper still, so the shear force is considerably greater than the shear strength, and the block will very likely move. (1)
Variability Due to Rock Type
The strength of the materials on slopes can vary widely. Solid rocks tend to be strong, but there is a very wide range of rock strength. If we consider just the strength of the rocks, and ignore issues like fracturing and layering, then most crystalline rocks—like granite, basalt, or gneiss—are very strong, while some metamorphic rocks—like schist—are moderately strong. Sedimentary rocks have variable strength. Some limestones are strong, most sandstone and conglomerate are moderately strong, and some sandstone and all shale are quite weak. Unconsolidated sediments are generally weaker than sedimentary rocks because they are not cemented and, in most cases, have not been significantly compressed by overlying materials.
Fractures, metamorphic foliation, or bedding can significantly reduce the strength of a body of rock, and in the context of mass wasting, this is most critical if the planes of weakness are parallel to the slope and least critical if they are perpendicular to the slope. This is illustrated in Figure 11.1.2. At locations A and B the bedding is nearly perpendicular to the slope and the situation is relatively stable. At location D the bedding is nearly parallel to the slope and the situation is quite unstable. At location C the bedding is nearly horizontal and the stability is intermediate between the other two extremes. (1)
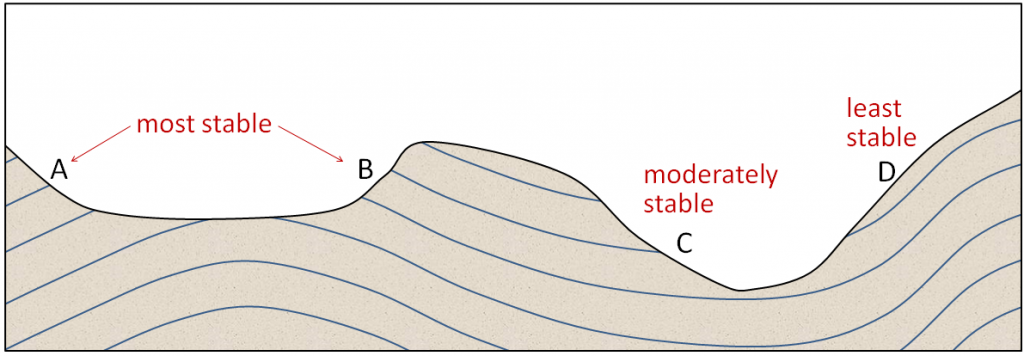
Affects of Water
Apart from the type of material on a slope, the amount of water that the material contains is the most important factor controlling its strength. This is especially true for unconsolidated materials, but it also applies to bodies of rock. Unconsolidated sediments tend to be strongest when they are moist because the small amounts of water at the grain boundaries hold the grains together with surface tension. Dry sediments are held together only by the friction between grains, and if they are well sorted or well rounded, or both, that cohesion is weak. Saturated sediments tend to be the weakest of all because the large amount of water actually pushes the grains apart, reducing the mount friction between grains. This is especially true if the water is under pressure. (1)
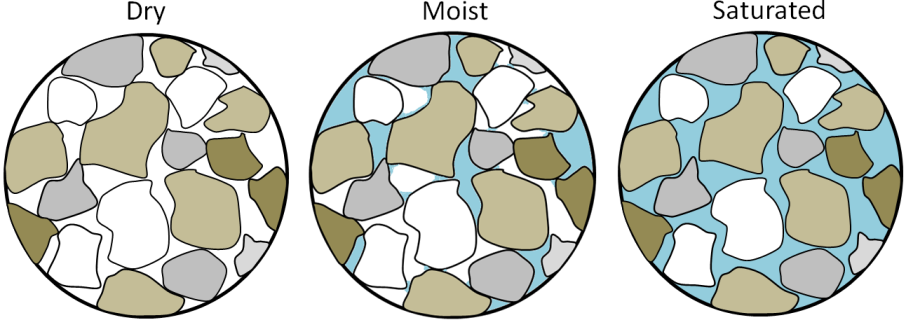
Digging Deeper: Sand, Water, and the Angle of Repose
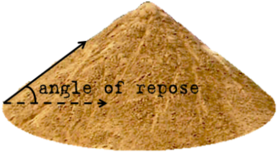
If you’ve ever been to the beach, you’ll already know that sand behaves differently when it’s dry than it does when it’s wet, but it’s worth taking a systematic look at the differences in its behavior. Find about half a cup of clean, dry sand (or get some wet sand and dry it out), and then pour it from your hand onto a piece of paper. You should be able to make a cone-shaped pile that has a slope of around 30°. If you pour more sand on the pile, it will get bigger, but the slope should remain the same. This is called the angle of repose, or the steepest angle formed by a certain loose material. Now add some water to the sand so that it is moist. An easy way to do this is to make it completely wet and then let the water drain away for a minute. You should be able to form this moist sand into a steep pile (with slopes of around 80°). The angle of repose increases, because the sediments are more cohesive in water. Finally, put the same sand into a cup and fill the cup with water so the sand is just covered. Swirl it around so that the sand remains in suspension, and then quickly tip it out onto a flat surface (best to do this outside). It should spread out over a wide area, forming a pile with a slope of only a few degrees. The angle of repose is less with additional water.
Water will also reduce the strength of solid rock, especially if it has fractures, bedding planes, or clay-bearing zones. All clay minerals will absorb a little bit of water, and this reduces their strength. The clays can absorb a lot of water, and that water pushes the sheets apart at a molecular level and makes the mineral swell. Water can also significantly increase the mass of the material on a slope. In Figure 7.1.1b, an increase in the shear force could easily be enough to tip the balance between shear force and shear strength. (1)
Triggers of Mass Wasting
In the previous section, we talked about the shear force and the shear strength of materials on slopes, and about factors that can reduce the shear strength. Shear force is primarily related to slope angle, and this does not change quickly. But shear strength can change quickly for a variety of reasons, and events that lead to a rapid reduction in shear strength are considered to be triggers for mass wasting.
An increase in water content is the most common mass-wasting trigger. This can result from rapid melting of snow or ice, heavy rain, or some type of event that changes the pattern of water flow on the surface. Rapid melting can be caused by a dramatic increase in temperature (e.g., in spring or early summer) or by a volcanic eruption. Heavy rains are typically related to major storms. Changes in water flow patterns can be caused by earthquakes, previous slope failures that dam up streams, or human structures that interfere with runoff (e.g., buildings, roads, or parking lots). In some cases, a decrease in water content can lead to failure. This is most common with clean sand deposits, which lose strength when there is no water to hold the grains together. Freezing and thawing can also trigger some forms of mass wasting. More specifically, the thawing can release a block of rock that was attached to a slope by a film of ice. (1)
One other process that can weaken a body of rock or sediment is shaking. The most obvious source of shaking is an earthquake, but shaking from highway traffic, construction, or mining will also do the job. Several deadly mass-wasting events (including snow avalanches) were triggered by the M7.8 earthquake in Nepal in April 2015.
Of course, a combination of triggers can have devastating effects. Saturation with water and then seismic shaking led to the occurrence of thousands of slope failures in the Sapporo area of Hokkaido, Japan in September 2018, as shown on Figure 11.1.5. The area was drenched with rain from tropical storm Jebi on September 4th. On September 6th it was shaken by a M6.6 earthquake which triggered debris flows in the water-saturated volcanic materials on steep slopes. There were 41 deaths related to the slope failures.
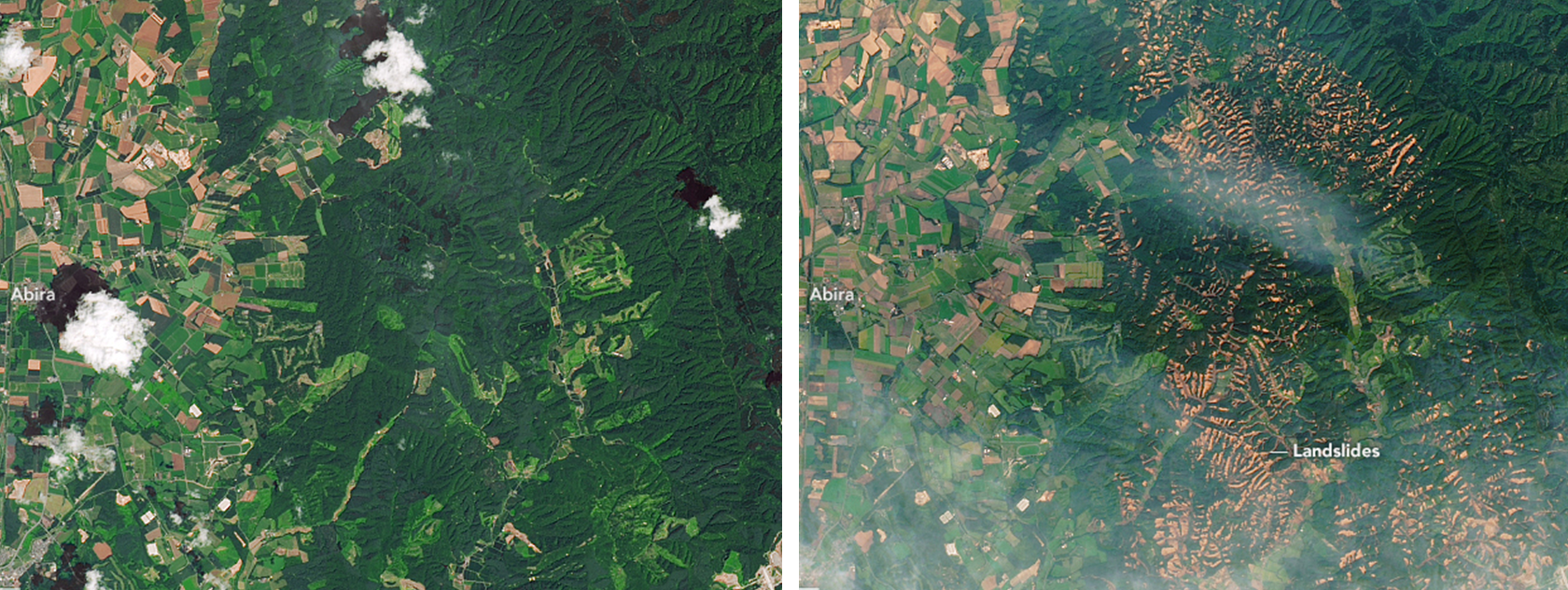
An over steepened slope may also trigger landslides. Slopes can be made excessively steep by natural processes of erosion or when humans modify the landscape for building construction. An example of how a slope may be over steepened during development occurs where the bottom of the slope is cut into, perhaps to build a road or level a building lot, and the top of the slope is modified by depositing excavated material from below. If done carefully, this practice can be very useful in land development, but in some cases, this can result in devastating consequences. (3)
Backyard Geology: Black Canyon Landslide

Arizona has over 8,000 documented landslide features, but they have occurred due to a variety of reasons. Figure 7.1.6 shows a small (less than 0.1 square miles) landslide that occurred near Black Canyon City, Arizona. This feature shows tension cracks. The portion labeled “head scarp” is the part of the butte that has stayed in place and is now exposed from the mass wasting event. The entire area is a series of flat-topped mesas comprised of lava flows and overlying shale, mudstone, and clays. The slide at Black Canyon City has shown recent movement and is still observed and studied!
***See 11.5 for Text and Media Attributions
The theory that the outer layer of the Earth (the lithosphere) is broken in several plates, and these plates move relative to one another, causing the major topographic features of Earth (e.g. mountains, oceans) and most earthquakes and volcanoes.
the process in which a material is worn away by a stream of liquid (water) or air, often due to the presence of abrasive particles in the stream
force acting in a direction parallel to a surface
a force that is perpendicular to the surface an object contacts
A felsic, intrusive rock with coarse-grained texture. Granite composes mountain cores and can be found on and within the continental crust.
an igneous rock that is mafic and fine-grained. Basalt is dark and makes up the majority of the oceanic crust.
A foliated, high-grade metamorphic rock distinguished by its strong preferred orientation, or banding, of light and dark minerals.
Rocks that form when any type of preexisting rock is warped or transformed under elevated temperatures and pressures.
A strongly foliated, medium-grade metamorphic rock with large, leaf-like grains of mica and sometimes red garnets.
rocks that cement together from weathering products, either from sediments or chemical ions in water.
An organic or chemical sedimentary rock that is primarily composed of calcium carbonate (CaCO3). Limestone is a subgroup of rocks that includes chalk, coquina, and fossiliferous limestone.
A type of clastic sedimentary rock that is composed of sand-sized clasts.
A type of clastic sedimentary rock that consists rounded pebble or cobble-sized clasts that are cemented together with finer grained sediment.
A clastic sedimentary rock made of very fine-grained sediments such as muds, clays, and silts.
The arrangement of thin layers or repetitive structures in metamorphic rocks that results from the uneven applications of heat and pressure within the Earth's surface.
The maximum slope, measured in degrees from a flat surface, at which loose solid material will remain in place without sliding.
A group of silicate minerals that typically forms after existing rocks and minerals, such as feldspars, are chemically altered by water.
pulling apart