10.3 Measuring and Locating Earthquakes
Focus and Epicenter
The point of initial breaking or rupturing, where the displacement of rocks occurs along the rupture surface, is called the focus. The focus is always at some depth below the ground surface in the crust, and not at the surface, as shows in Figure 10.3.1. From the focus, the displacement propagates up, down, and laterally along the fault plane. The displacement produces shock waves, creating seismic waves. Notice that the location of the fault scarp may be a distance from the epicenter. The larger the displacement and the further it propagates, the more significant the seismic waves and ground shaking. More shaking is usually the result of more seismic energy released. The epicenter is the location on the Earth’s surface vertically above the point of rupture (focus). The epicenter is also the location that most news reports give because it is the center of the area where people are affected. The focus is the point along the fault plane from which the seismic waves spread outward. (4)

Seismic Waves
Seismic waves are an expression of the elastic energy released after an earthquake that travel either along the Earth’s surface (surface waves) or throughout the Earth’s interior (body waves). When seismic energy is released, the first waves to propagate out are body waves that pass through the planet’s interior. Body waves include primary waves (P waves) and secondary waves (S waves).
Body Waves
Primary waves are the fastest seismic waves. They move through the rock via compression, very much like sound waves move through the air. Particles of rock move forward and back during the passage of the P waves. Primary waves can travel through both fluids and solids. Secondary waves travel slower and follow primary waves, propagating as shear waves. Particles of rock move from side to side during the passage of S waves. Due to this motion, secondary waves cannot travel through liquids, plasma, or gas.
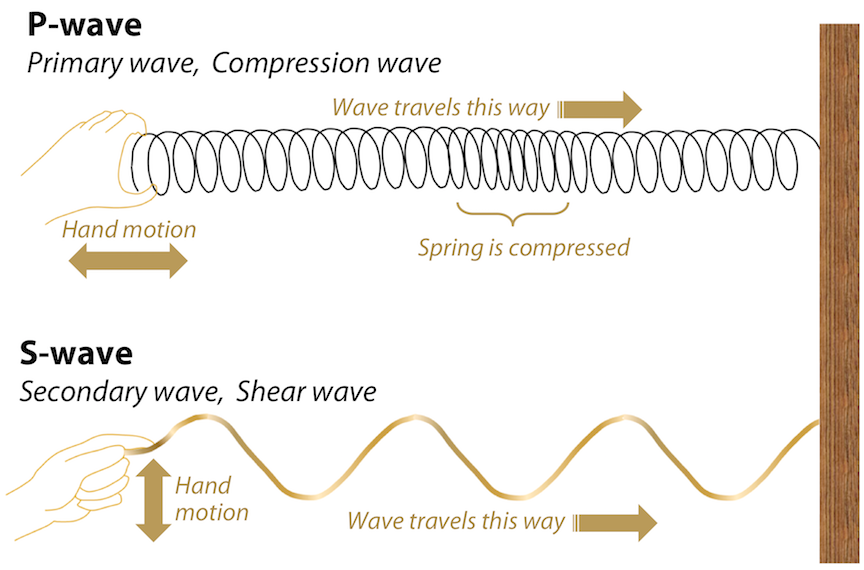
Because the interior of the Earth is not made of a single, homogenous substance, body waves do not travel uniformly through it. Seismic waves reflect (bounce), refract (bend), and speed up with depth as they encounter various boundaries and densities within the Earth. This behavior typically happens at the boundaries between the crust, mantle, and core. Because S waves cannot move through a liquid, they are blocked by the liquid outer core, creating a shadow zone on the opposite side of the planet to the earthquake source. P waves also have a shadow zone, created from areas that occur due to the pattern of refraction (Figure 10.3.3).
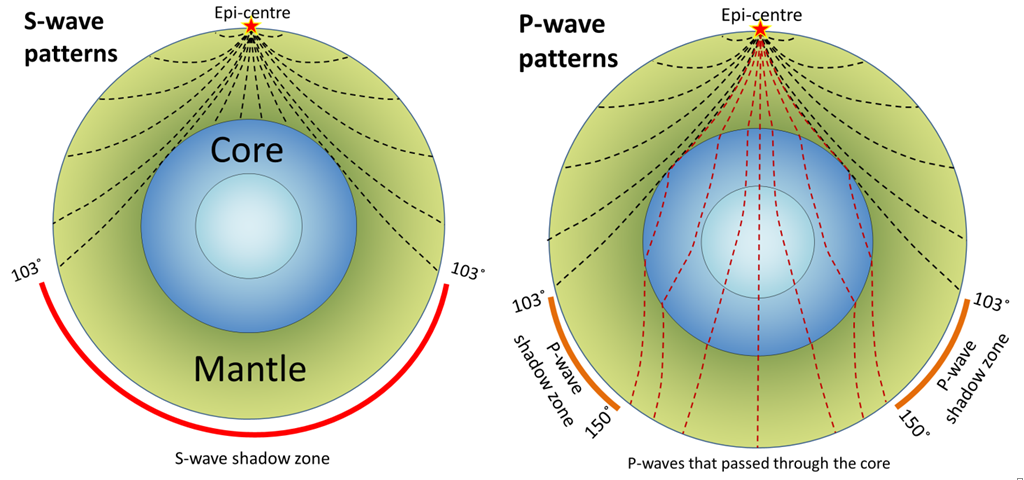
Surface Waves
Surface waves are slower moving seismic wave, and are produced when body waves reach the Earth’s surface. Two distinctly different waves are created spread out along the surface of the earth until they dissipate. Love waves produce horizontal ground shaking and, ironically from their name, are the most destructive. Rayleigh waves produce an elliptical motion of points on the surface, with longitudinal dilation and compression, like ocean waves. However, with Raleigh waves, rock particles move in a direction opposite to that of water particles in ocean waves. (4)
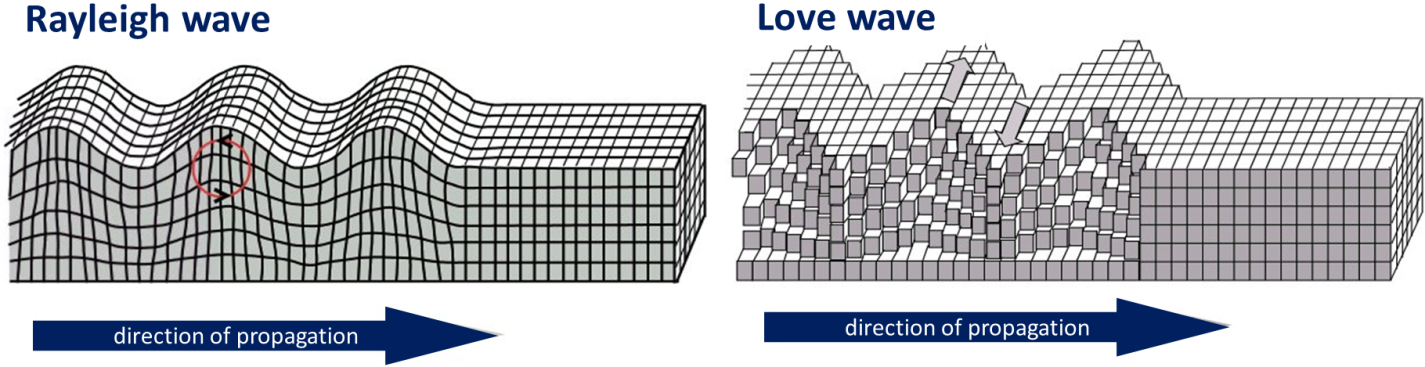
Seismographs
Seismographs are instruments used to measure seismic waves. They measure the vibration of the ground using pendulums or springs. The seismograph principle involves mounting a recording device solidly to the earth and suspending a pen or writing instrument above it on a spring or pendulum. As the ground shakes, the suspended pen records the shaking on the recording device. The graph resulting from measurements of a seismograph is a seismogram. Seismographs of the early 20th century were mostly springs or pendulums with pens on them that wrote on a rotating drum of paper (Figure 5.3.5). Digital ones now use magnets and wire coils to measure ground motion. Typical seismograph arrays measure vibrations in three directions: north-south (x), east-west (y), and up-down (z). (4)

Body waves and surface waves are recorded on seismograms, with P waves arriving first, S waves second, and surface waves following.
Surface waves do lose energy quickly, so they are not measured at great distances from the focus. Seismograph technology across the globe record the arrival of seismic waves from each earthquake at many station sites. The distance to the epicenter can be determined by comparing arrival times of the P and S waves. Electronic communication among seismic stations and connected computers used to make calculations mean that locations of earthquakes and news reports about them are generated quickly in the modern world. (4)

Locating Earthquakes
Each seismograph gives the distance from that station to the earthquake epicenter. Three or more seismograph stations are needed to locate the epicenter of an earthquake through triangulation . Using the arrival-time difference from the first P wave to the first S wave, one can determine the distance from the epicenter, but not the direction. The distance from the epicenter to each station can be plotted as a circle, the distance being equal to the circle’s radius. The place where the circles intersect demarks the epicenter (Figure 10.3.7). This method also works in three dimensions with spheres and multi-axis seismographs to locate not only the epicenter but also the depth of the earthquake’s focus.
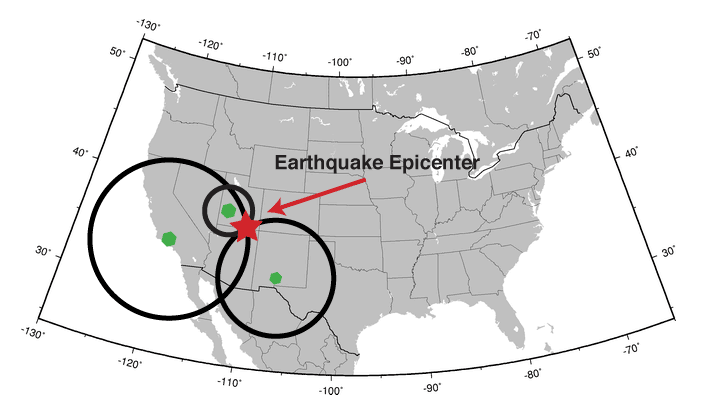
Seismograph Network
The International Registry of Seismograph Stations lists more than 26,000 seismographs on the planet. Seismologists can use and compare data from sets of multiple seismometers dispersed over a wide area, a seismograph network. By collaborating, scientists can map the inside of the earth’s properties, detect large explosive devices, and predict tsunamis. The Global Seismograph Network, a set of worldwide linked seismographs that distribute real-time data electronically, consists of more than 150 stations that meet specific design and precision standards. The Global Seismograph Network helps the Comprehensive Nuclear-Test-Ban Treaty Organization monitor for nuclear tests. The USArray is a network of hundreds of permanent and transportable seismographs within the United States. The USArray is being used to map the subsurface through a passive collection of seismic waves created by earthquakes. (4)
Determining Earthquake Size
There are typically two questions that are asked immediately after an earthquake: Where did it occur? How big was it? Previously we discussed the science behind determining where an earthquake occurred. Earthquake size is usually described by either their intensity or their magnitude. Intensity describes the severity of an earthquake in terms of the effects of the Earth’s surface on society. Initially, all earthquakes were described using intensity scales, but these scales are inconsistent and biased depending on where the event occurred. Magnitude, by contrast, describes the amount of energy released at the focus of an earthquake, regardless of how it is felt or damage that occurs. Over time, several magnitude scales have been created, and earthquakes today are given magnitude (M) numbers to describe them.
Modified Mercalli-Intensity Scale
There are many scales for intensity, but the United States commonly uses the Modified Mercalli scale. The Modified Mercalli Intensity Scale is a qualitative scale (I-XII) of the intensity of ground shaking based on damage to structures and people’s perceptions (Figure 10.3.8). This scale can vary depending on the location and population density (urban vs. rural). It was also used for historical earthquakes, which occurred before quantitative measurements of magnitude could be made. The Modified Mercalli Intensity maps show where the damage is most severe based on questionnaires sent to residents, newspaper articles, and reports from assessment teams. Recently, USGS has used the internet to help gather data more quickly.
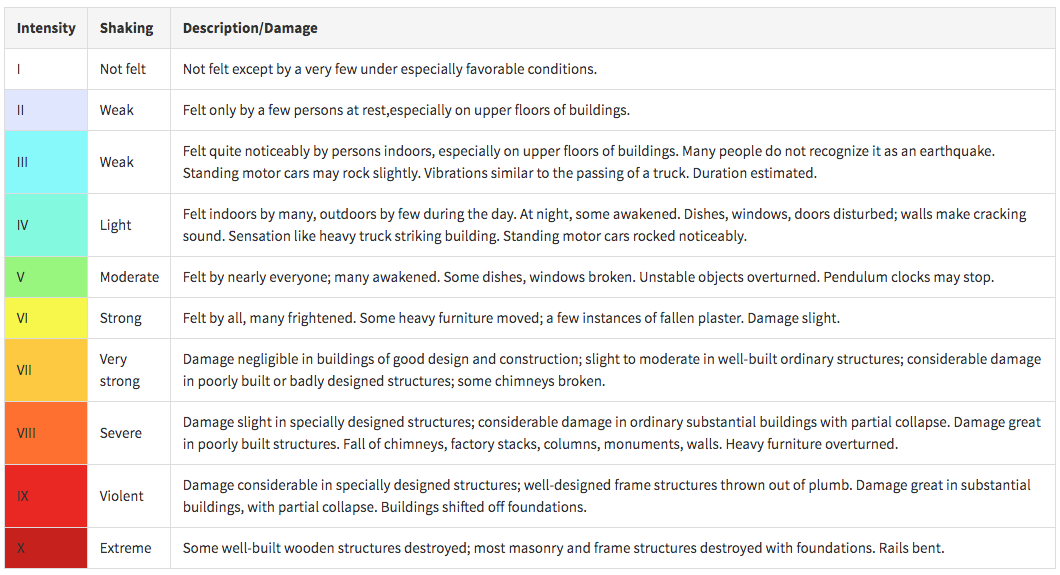
Richter Scale
Magnitude is the most common measure of earthquake size, as it determines the size of the earthquake at the focus and is the same number no matter where you are or what the shaking may feel like. Though outdated and no longer used, the Richter scale is the most well-known magnitude scale devised for an earthquake and was the first one developed by Charles Richter at CalTech. This was the magnitude scale used historically by early seismologists. The Richter scale magnitude is determined from measurements on a seismogram. Magnitudes on the Richter scale are based on measurements of the maximum amplitude of the needle trace measured on the seismogram and the arrival time difference of S and P waves, which gives the distance to the earthquake. (4)
The Richter scale is a logarithmic scale, based on powers of 10. The amplitude of the seismic wave recorded on the seismogram is ten times greater for each increase of 1 unit on the Richter scale. That means a magnitude six earthquake shakes the ground ten times more than a magnitude 5. However, the actual energy released for each 1-unit magnitude increase is 32 times greater. That means energy released for a magnitude six earthquake is 32 times greater than a magnitude 5 earthquake. The Richter scale was developed for distances appropriate for earthquakes in Southern California and on seismograph machines in use there. Its applications to more considerable distances and massive earthquakes are limited. Therefore, most agencies no longer use Richter’s methods to determine the magnitude but generate a quantity called the Moment Magnitude, which is more accurate for large earthquakes measured at the seismic array across the earth. As numbers, the moment magnitudes are comparable to the magnitudes of the Richter Scale. The media still often give magnitudes as Richter Magnitude even though the actual calculation was of moment magnitude. (4)
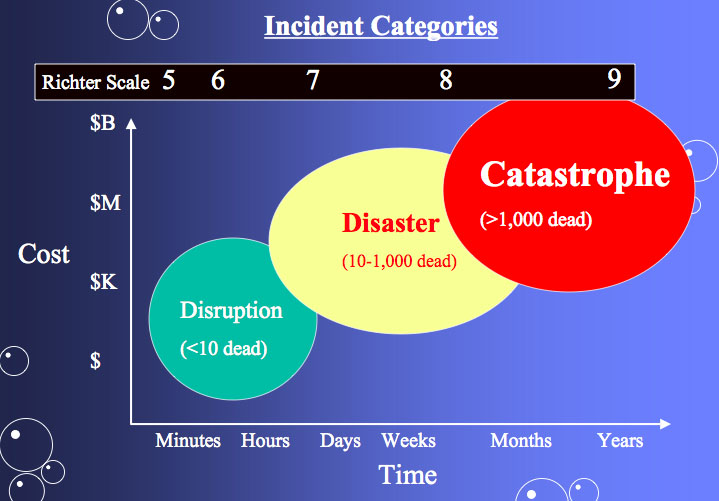
Moment Magnitude Scale
The Moment Magnitude Scale depicts the absolute size of earthquakes, comparing information from multiple locations and using a measurement of actual energy released calculated from the cross-sectional area of rupture, amount of slippage, and the rigidity of the rocks. Because of the unique geologic setting of each earthquake and because the rupture area is often hard to measure, estimates of moment magnitude can take days to months to calculate. (4)
Like the Richter magnitude, the moment magnitude scale is logarithmic. Both scales are used in tandem because the estimates of magnitude may change after a quake. The Richter scale is used as a quick determination immediately following the quake (and is usually reported in news accounts), and the moment magnitude is calculated days to months later. The magnitude values of the two magnitudes are approximately equal except for massive earthquakes.
Backyard Geology: Seismic Network in Arizona
The Arizona Geologic Survey (AZGS), working with geoscientists at Northern Arizona University (NAU), built and maintains a statewide seismic monitoring network to record earthquakes and to look for patterns of seismic activity. In 2017, the Arizona Broadband Seismic Network (ABSN) was expanded from 8 to 13 stations, strategically located to provide statewide coverage and to capture more of the seismicity throughout Arizona. More than 3,000 historic (1852-2017) earthquake events have been recorded and compiled.
***See 10.6 for Text and Media Attributions
the point of initial breaking or rupturing where the displacement of rocks occurs
The surface along which there is slip during an earthquake, also called the rupture surface
location on the Earth’s surface vertically above the point of rupture (focus)
Seismic waves that travel at the Earth's surface.
seismic waves that travel through the Earth's interior
Shear seismic wave that travels throughout the Earth's interior
The thin, outermost layer of Earth composed of rigid rock, which is home to all known life on the planet.
A hot interior layer of solid rock between the crust and core that is capable of plastic flow. The mantle is the largest layer of Earth.
The extremely hot center layer within Earth, which is composed mainly of iron and nickel.
instruments used to measure seismic waves
The graph resulting from measurements of a seismograph
An instrument that measures and records seismic waves caused by geologic activity in the Earth's interior. The graphical product of a seismograph recording is called a seismogram.
The intensity is a number (written as a Roman numeral) describing the severity of an earthquake in terms of its effects on the earth's surface and on humans and their structures.