5.1 Volcano Eruption Basics
In 1980, Mount St. Helens blew up in the costliest and deadliest volcanic eruption in United States history. The eruption killed 57 people, destroyed 250 homes, and swept away 47 bridges. Mount St. Helens today still has minor earthquakes and eruptions, and now has a horseshoe-shaped crater with a lava dome inside. The dome is made of pb_glossary id=”1812″]viscous[/pb_glossary] lava that oozes into place [1].
Volcanoes do not always erupt in the same way. Each volcanic eruption is unique, differing in size, style, and composition of the erupted material. One key to what makes the eruption unique is the chemical composition of the magma that feeds a volcano, which determines (1) the eruption style, (2) the type of volcanic cone that forms, and (3) the composition of rocks that are found at the volcano.
Volcanoes with a mafic composition will typically not have very explosive eruptions, but the lava erupted will be fast moving. This mafic lava often moves down mountainsides and cools rapidly into unique textures that are either ropey called “Pahoehoe” or rough and rocky called “A’a”.
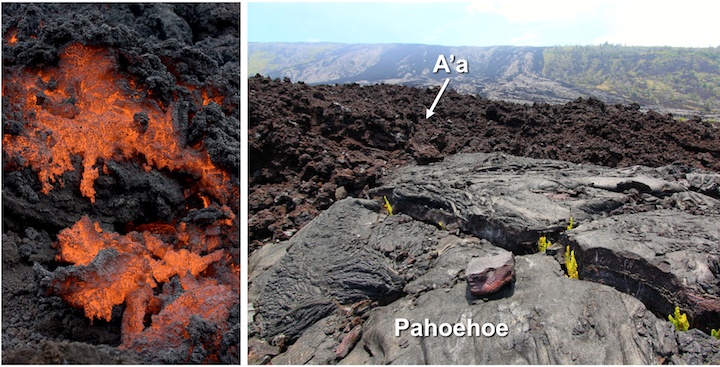
Felsic magma is very viscous, and it does not flow smoothly. Most felsic magma will stay deeper in the crust and will cool to form intrusive igneous rocks such as granite and diorite. If felsic magma rises into a magma chamber, it may be too viscous to move, so it tends to get stuck.
However, intermediate magma is also highly viscous, and it contains dissolved volatile gases. These gases become trapped by the magma, and the magma chamber begins to build in pressure. When the magma finally can erupt as lava, it does so very violently and explosively, as we have seen at Mount St. Helens.
***See 5.8 for Text and Media Attributions
As previously discussed in section 3.9, a rock is a collection of consolidated minerals. Rocks are grouped into three main categories based on how they form:
- Igneous: formed from the cooling and crystallization of molten rock.
- Sedimentary: formed when weathered fragments of other rocks are buried, compressed, and cemented together, or when minerals precipitate directly from solution
- Metamorphic: formed by alteration (due to heat, pressure, and/or chemical action) of a pre-existing igneous or sedimentary rock
The focus of this chapter is on Igneous rocks, though it is important to remember this is just a piece of the rock cycle.
Learning Objectives
After carefully reading this chapter, completing the exercises within it, and answering the questions at the end, you should be able to:
- Describe the rock cycle and the types of processes that lead to the formation of igneous, sedimentary, and metamorphic rocks, and explain why there is an active rock cycle on Earth.
- Explain the concept of partial melting and describe the geological processes that lead to melting.
- Describe, in general terms, the range of chemical compositions of magmas.
- Discuss the processes that take place during the cooling and crystallization of magma, and the typical order of crystallization according to the Bowen reaction series.
- Explain how magma composition can be changed by fractional crystallization and partial melting of the surrounding rocks.
- Apply the criteria for igneous rock classification based on mineral proportions.
- Describe the origins of phaneritic, porphyritic, and pegmatitic rock textures.
- Identify plutons on the basis of their morphology and their relationships to the surrounding rocks.
- Explain the origin of a chilled margin.
molten rock that can be found beneath the Earth's surface.
Originating from an iron and magnesium-rich magma/lava composition.
originating from a feldspar and silica-rich magma/lava composition.
coarse-grained igneous rock texture with visible crystals within the matrix.
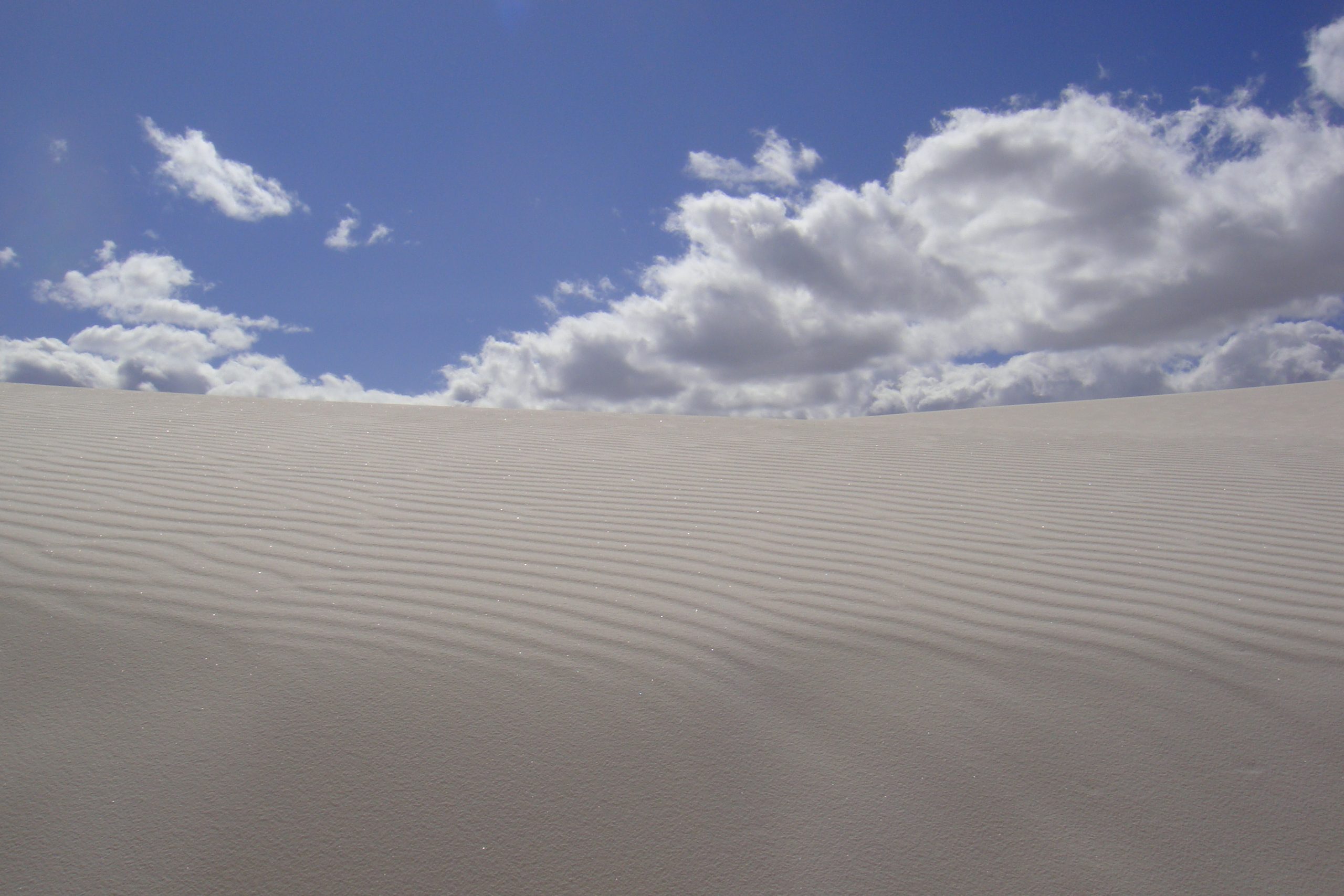
13 Deserts
KEY CONCEPTS
By the end of this chapter, students should be able to:
- Explain the defining characteristic of a desert and distinguish between the three broad categories of deserts
- Explain how geographic features, <span aria-describedby="tt" class="glossaryLink" data-cmtooltip="
latitudeThe measure of degrees north or south from the equator, which has a latitude of 0 degrees. The Earth's north and south poles have latitudes of 90 degrees north and south, respectively.
">latitude, atmospheric circulation, and Coriolis Effect influence where deserts are located
- List the primary desert <span aria-describedby="tt" class="glossaryLink" data-cmtooltip="
weatheringBreaking down rocks into small pieces by chemical or mechanical means.
">weathering and <span aria-describedby="tt" class="glossaryLink" data-cmtooltip="
erosionThe transport and movement of weathered sediments.">erosion processes
- Identify desert landforms
- Explain how desert landforms are formed by <span aria-describedby="tt" class="glossaryLink" data-cmtooltip="
erosionThe transport and movement of weathered sediments.
">erosion and <span aria-describedby="tt" class="glossaryLink" data-cmtooltip="
depositionSediment gathering together and collecting, typically in a topographic low point.">deposition
- Describe the main types of sand duneA large pile of sediment, deposited perpendicular to flow. Internal bedding in dunes dips toward flow direction (i.e. cross bedding). Formed in the upper part of the lower flow regime.">dunes and the conditions that form them
- Identify the main features of the <span aria-describedby="tt" class="glossaryLink" data-cmtooltip="
basin and rangeTerm for the extensional tectonic province that extends from California's Sierra Nevada Mountains in the west, to Utah's Wasatch Mountains to the east, to southern Oregon and Idaho to the north, to northern Mexico to the south. Known as a wide rift, as each graben 'basin,' bounded by horst 'ranges.' Each set of horsts with a graben has some individual extension, adding up to the overall rifting.
">Basin and Range desert (United States)
Approximately 30% of the Earth’s <span aria-describedby="tt" class="glossaryLink" data-cmtooltip="
">terrestrial surface consists of deserts, which are defined as locations of low <span aria-describedby="tt" class="glossaryLink" data-cmtooltip="
">precipitation. While <span aria-describedby="tt" class="glossaryLink" data-cmtooltip="
">temperature extremes are often associated with deserts, they do not define them. Deserts exhibit extreme temperatures because of the lack of moisture in the <span aria-describedby="tt" class="glossaryLink" data-cmtooltip="
">atmosphere, including low humidity and scarce cloud cover. Without cloud cover, the Earth’s surface absorbs more of the Sun’s energy during the day and emits more heat at night.
Deserts are not randomly located on the Earth’s surface. Many deserts are located at latitudes between 15° and 30° in both hemispheres and at both the North and South Poles, created by prevailing wind circulation in the <span aria-describedby="tt" class="glossaryLink" data-cmtooltip="
">atmosphere. Sinking, dry air currents occurring at 30° north and south of the equator produce <span aria-describedby="tt" class="glossaryLink" data-cmtooltip="
">trade winds that create deserts like the African Sahara and Australian Outback.
Another type of desert is found in the rain shadow created from prevailing winds blowing over mountain ranges. As the wind drives air up and over mountains, atmospheric moisture is released as snow or rain. Atmospheric pressure is lower at higher elevations, causing the moisture-laden air to cool. Cool air holds less moisture than hot air, and <span aria-describedby="tt" class="glossaryLink" data-cmtooltip="
">precipitation occurs as the wind rises up the mountain. After releasing its moisture on the windward side of the mountains, the dry air descends on the leeward or downwind side of the mountains to create an arid region with little <span aria-describedby="tt" class="glossaryLink" data-cmtooltip="
">precipitation called a rain shadow. Examples of rain-shadow deserts include the Western Interior Desert of North America and <span aria-describedby="tt" class="glossaryLink" data-cmtooltip="
">Atacama Desert of Chile, which is the Earth’s driest, warm desert.
Finally, <span aria-describedby="tt" class="glossaryLink" data-cmtooltip="
">polar deserts, such as vast areas of the Antarctic and Arctic, are created from sinking cold air that is too cold to hold much moisture. Although they are covered with ice and snow, these deserts have very low average annual <span aria-describedby="tt" class="glossaryLink" data-cmtooltip="
">precipitation. As a result, Antarctica is Earth’s driest <span aria-describedby="tt" class="glossaryLink" data-cmtooltip="
">continent.
13.1 The Origin of Deserts
13.1.1 Atmospheric circulation
Geographic location, atmospheric circulation, and the Earth’s rotation are the primary causal factors of deserts. Solar energy converted to heat is the engine that drives the circulation of air in the <span aria-describedby="tt" class="glossaryLink" data-cmtooltip="
">atmosphere and water in the oceans. The strength of the circulation is determined by how much energy is absorbed by the Earth’s surface, which in turn is dependent on the average position of the Sun relative to the Earth. In other words, the Earth is heated unevenly depending on <span aria-describedby="tt" class="glossaryLink" data-cmtooltip="
">latitude and <span aria-describedby="tt" class="glossaryLink" data-cmtooltip="
">angle of incidence. <span aria-describedby="tt" class="glossaryLink" data-cmtooltip="
">Latitude is a line circling the Earth parallel to the equator and is measured in degrees. The equator is 0° and the North and South Poles are 90° N and 90° S respectively (see the diagram of generalized atmospheric circulation on Earth). <span aria-describedby="tt" class="glossaryLink" data-cmtooltip="
">Angle of incidence is the angle made by a ray of sunlight shining on the Earth’s surface. Tropical zones are located near the equator, where the <span aria-describedby="tt" class="glossaryLink" data-cmtooltip="
">latitude and <span aria-describedby="tt" class="glossaryLink" data-cmtooltip="
">angle of incidence are close to 0°, and receive high amounts of solar energy. The poles, which have latitudes and angles of incidence approaching 90°, receive little or almost no energy.
The figure shows the generalized air circulation within the <span aria-describedby="tt" class="glossaryLink" data-cmtooltip="
">atmosphere. Three cells of circulating air span the space between the equator and poles in both hemispheres, the <span aria-describedby="tt" class="glossaryLink" data-cmtooltip="
">Hadley Cell, the Ferrel or Midlatitude Cell, and the <span aria-describedby="tt" class="glossaryLink" data-cmtooltip="
">Polar Cell. In the <span aria-describedby="tt" class="glossaryLink" data-cmtooltip="
">Hadley Cell located over the tropics and closest to the equatorial belt, the sun heats the air and causes it to rise. The rising air cools and releases its contained moisture as tropical rain. The rising dried air spreads away from the equator and toward the north and south poles, where it collides with dry air in the Ferrel Cell. The combined dry air sinks back to the Earth at 30° <span aria-describedby="tt" class="glossaryLink" data-cmtooltip="
">latitude. This sinking drier air creates belts of predominantly high pressure at approximately 30° north and south of the equator, called the horse latitudes. Arid zones between 15o and 30o north and south of the equator thus exist within which desert conditions predominate. The descending air flowing north and south in the Hadley and Ferrel cells also creates prevailing winds called <span aria-describedby="tt" class="glossaryLink" data-cmtooltip="
">trade winds near the equator, and <span aria-describedby="tt" class="glossaryLink" data-cmtooltip="
">westerlies in the temperate zone. Note the arrows indicating general directions of winds in these zones.
Other deserts, like the <span aria-describedby="tt" class="glossaryLink" data-cmtooltip="
">Great Basin Desert that covers parts of Utah and Nevada, owe at least part of their origin to other atmospheric phenomena. The <span aria-describedby="tt" class="glossaryLink" data-cmtooltip="
">Great Basin Desert, while somewhat affected by sinking air effects from global circulation, is a rain-shadow desert. As westerly moist air from the Pacific rises over the Sierra Nevada and other mountains, it cools and loses moisture as condensation and <span aria-describedby="tt" class="glossaryLink" data-cmtooltip="
">precipitation on the upwind or rainy side of the mountains.
One of the driest places on Earth is the <span aria-describedby="tt" class="glossaryLink" data-cmtooltip="
">Atacama Desert of northern Chile. The <span aria-describedby="tt" class="glossaryLink" data-cmtooltip="
">Atacama Desert occupies a strip of land along Chile’s <span aria-describedby="tt" class="glossaryLink" data-cmtooltip="
">coast just north of <span aria-describedby="tt" class="glossaryLink" data-cmtooltip="
">latitude 30°S, at the southern edge of the trade-wind belt. The desert lies west of the Andes Mountains, in the rain shadow created by prevailing <span aria-describedby="tt" class="glossaryLink" data-cmtooltip="
">trade winds blowing west. As this warm moist air crossing the Amazon <span aria-describedby="tt" class="glossaryLink" data-cmtooltip="
">basin meets the eastern edge of the mountains, it rises, cools, and precipitates much of its water out as rain. Once over the mountains, the cool, dry air descends onto the <span aria-describedby="tt" class="glossaryLink" data-cmtooltip="
">Atacama desert. Onshore winds from the Pacific are cooled by the Peru (Humboldt) ocean current. This super-cooled air holds almost no moisture and, with these three factors, some locations in the <span aria-describedby="tt" class="glossaryLink" data-cmtooltip="
">Atacama Desert have received no measured <span aria-describedby="tt" class="glossaryLink" data-cmtooltip="
">precipitation for several years. This desert is the driest, non-polar location on Earth.
Notice in the figure that the polar regions are also areas of predominantly high pressure created by descending cold dry air, the <span aria-describedby="tt" class="glossaryLink" data-cmtooltip="
">Polar Cells. As with the other cells, cold air, which holds much less moisture than warm air, descends to create <span aria-describedby="tt" class="glossaryLink" data-cmtooltip="
">polar deserts. This is why historically, land near the north and south poles has always been so dry.
13.1.2 Coriolis Effect
The Earth rotates toward the east where the sun rises. Think of spinning a weight on a string around your head. The speed of the weight depends on the length of the string. The speed of an object on the rotating Earth depends on its horizontal distance from the Earth’s <span aria-describedby="tt" class="glossaryLink" data-cmtooltip="
">axis of rotation. Higher latitudes are a smaller distance from the Earth’s rotational <span aria-describedby="tt" class="glossaryLink" data-cmtooltip="
">axis, and therefore do not travel as fast eastward as lower latitudes that are closer to the equator. When a fluid like air or water moves from a lower <span aria-describedby="tt" class="glossaryLink" data-cmtooltip="
">latitude to a higher <span aria-describedby="tt" class="glossaryLink" data-cmtooltip="
">latitude, the fluid maintains its momentum from moving at a higher speed, so it will travel relatively faster eastward than the Earth beneath at the higher latitudes.
Since each hemisphere has three atmospheric cells moving respectively north and south relative to the Earth beneath them, the Coriolis effect deflects these moving air masses to the right in the Northern Hemisphere and to the left in the Southern Hemisphere. The Coriolis effect also deflects moving masses of water in the ocean currents.
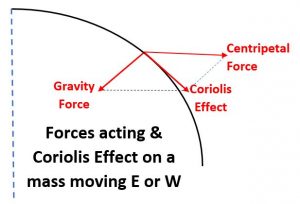
\For example, in the northern hemisphere <span aria-describedby="tt" class="glossaryLink" data-cmtooltip="
">Hadley Cell, the lower altitude air currents are flowing south towards the equator. These are deflected to the right (or west) by the Coriolis effect. This deflected air generates the prevailing <span aria-describedby="tt" class="glossaryLink" data-cmtooltip="
">trade winds that European sailors used to cross the Atlantic Ocean and reach South America and the Caribbean Islands in their tall ships. This air movement is mirrored in the <span aria-describedby="tt" class="glossaryLink" data-cmtooltip="
">Hadley Cell in the southern hemisphere; the lower altitude air current flowing equatorward is deflected to the left, creating <span aria-describedby="tt" class="glossaryLink" data-cmtooltip="
">trade winds that blow to the northwest.
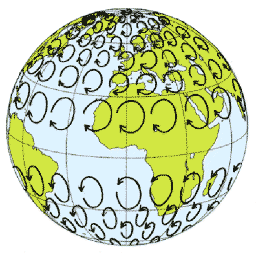
In the northern Mid-<span aria-describedby="tt" class="glossaryLink" data-cmtooltip="
">Latitude or Ferrel Cell, surface air currents flow from the horse latitudes (<span aria-describedby="tt" class="glossaryLink" data-cmtooltip="
">latitude 30°) toward the North Pole, and the Coriolis effect deflects them toward the east, or to the right, producing the zone of westerly winds. In the southern hemisphere Mid-<span aria-describedby="tt" class="glossaryLink" data-cmtooltip="
">Latitude or Ferrel Cell, the poleward flowing surface air is deflected to the left and flows southeast creating the Southern Hemisphere <span aria-describedby="tt" class="glossaryLink" data-cmtooltip="
">westerlies.
Another Coriolis-generated deflection produces the <span aria-describedby="tt" class="glossaryLink" data-cmtooltip="
">Polar Cells. At 60o north and south <span aria-describedby="tt" class="glossaryLink" data-cmtooltip="
">latitude, relatively warmer rising air flows poleward cooling and converging at the poles where it sinks in the polar high. This sinking dry air creates the <span aria-describedby="tt" class="glossaryLink" data-cmtooltip="
">polar deserts, the driest deserts on Earth. Persistence of ice and snow is a result of cold temperatures at these dry locations.
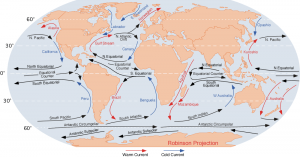
Artillerymen must take the Coriolis effect into account on ballistic trajectories when making long-distance targeting calculations. Geologists note how its effect on air and <span aria-describedby="tt" class="glossaryLink" data-cmtooltip="
">oceanic currents creates deserts in designated zones around the Earth as well as the surface currents in the ocean. The Coriolis effect causes the ocean gyreLarge circular ocean currents formed by global atmospheric circulation patters.">gyres to turn clockwise in the northern hemisphere and counterclockwise in the southern. It also affects <span aria-describedby="tt" class="glossaryLink" data-cmtooltip="
">weather by creating high-altitude, polar jet <span aria-describedby="tt" class="glossaryLink" data-cmtooltip="
">streams that sometimes push lobes of cold arctic air into the temperate zone, down to as far as <span aria-describedby="tt" class="glossaryLink" data-cmtooltip="
">latitude 30° from the usual 60°. It also causes low pressure systems and intense tropical storms to rotate counter-clockwise in the Northern Hemisphere and clockwise in the Southern Hemisphere.
Explanation of Coriolis Effect.
13.2 Desert weathering and erosion
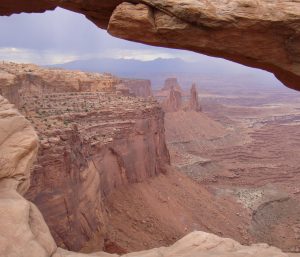
<span aria-describedby="tt" class="glossaryLink" data-cmtooltip="
">Weathering takes place in desert climates by the same means as other climates, only at a slower rate. While higher temperatures typically spur faster <span aria-describedby="tt" class="glossaryLink" data-cmtooltip="
">chemical weathering, water is the main agent of <span aria-describedby="tt" class="glossaryLink" data-cmtooltip="
">weathering, and lack of water slows both mechanical and <span aria-describedby="tt" class="glossaryLink" data-cmtooltip="
">chemical weathering. Low <span aria-describedby="tt" class="glossaryLink" data-cmtooltip="
">precipitation levels also mean less <span aria-describedby="tt" class="glossaryLink" data-cmtooltip="
">runoff as well as <span aria-describedby="tt" class="glossaryLink" data-cmtooltip="
">ice wedging. When <span aria-describedby="tt" class="glossaryLink" data-cmtooltip="
">precipitation does occur in the desert, it is often heavy and may result in <span aria-describedby="tt" class="glossaryLink" data-cmtooltip="
">flash floods in which a lot of material may be dislodged and moved quickly.
One unique <span aria-describedby="tt" class="glossaryLink" data-cmtooltip="
">weathering product in deserts is <span aria-describedby="tt" class="glossaryLink" data-cmtooltip="
">desert varnish. Also known as desert patina or rock rust, this is thin dark brown layers of clay <span aria-describedby="tt" class="glossaryLink" data-cmtooltip="
">minerals and iron and manganese <span aria-describedby="tt" class="glossaryLink" data-cmtooltip="
">oxides that form on very stable surfaces within arid environments. The exact way this material forms is still unknown, though cosmogenic and biologic mechanisms have been proposed.
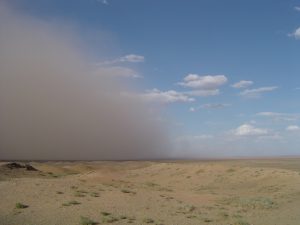
While water is still the dominant agent of <span aria-describedby="tt" class="glossaryLink" data-cmtooltip="
">erosion in most desert environments, wind is a notable agent of <span aria-describedby="tt" class="glossaryLink" data-cmtooltip="
">weathering and <span aria-describedby="tt" class="glossaryLink" data-cmtooltip="
">erosion in many deserts. This includes suspended <span aria-describedby="tt" class="glossaryLink" data-cmtooltip="
">sediment traveling in <span aria-describedby="tt" class="glossaryLink" data-cmtooltip="
">haboobs, or large dust storms, that frequent deserts. Deposits of windblown dust are called <span aria-describedby="tt" class="glossaryLink" data-cmtooltip="
">loess. <span aria-describedby="tt" class="glossaryLink" data-cmtooltip="
">Loess deposits cover wide areas of the midwestern United States, much of it from rock flour that melted out of the <span aria-describedby="tt" class="glossaryLink" data-cmtooltip="
">ice sheets during the last <span aria-describedby="tt" class="glossaryLink" data-cmtooltip="
">ice age. <span aria-describedby="tt" class="glossaryLink" data-cmtooltip="
">Loess was also blown from desert regions in the West. Possessing lower energy than water, wind transport nevertheless moves sand, silt, and dust. As noted in chapter 11, the load carried by a fluid (air is a fluid like water) is distributed among <span aria-describedby="tt" class="glossaryLink" data-cmtooltip="
">bedload and <span aria-describedby="tt" class="glossaryLink" data-cmtooltip="
">suspended load. As with water, in wind these components depend on wind velocity.
Sand size material moves by a process called <span aria-describedby="tt" class="glossaryLink" data-cmtooltip="
">saltation in which sand grains are lifted into the moving air and carried a short distance where they drop and splash into the surface dislodging other sand grains which are then carried a short distance and splash dislodging still others [zotpressInText item=”{J8N5GRPZ}” format=”%num%” brackets=”yes”].
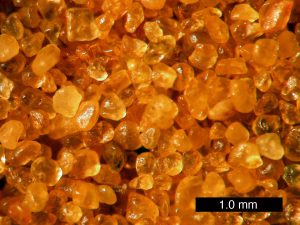
Since saltating sand grains are constantly impacting other sand grains, wind blown sand grains are commonly quite well rounded with frosted surfaces. <span aria-describedby="tt" class="glossaryLink" data-cmtooltip="
">Saltation is a cascading effect of sand movement creating a zone of wind blown sand up to a meter or so above the ground. This zone of saltating sand is a powerful <span aria-describedby="tt" class="glossaryLink" data-cmtooltip="
">erosive agent in which <span aria-describedby="tt" class="glossaryLink" data-cmtooltip="
">bedrock features are effectively sandblasted. The fine-grained <span aria-describedby="tt" class="glossaryLink" data-cmtooltip="
">suspended load is effectively sorted from the sand near the surface carrying the silt and dust into <span aria-describedby="tt" class="glossaryLink" data-cmtooltip="
">haboobs. Wind is thus an effective <span aria-describedby="tt" class="glossaryLink" data-cmtooltip="
">sorting agent separating sand and dust sized (≤70 µm) particles (See Chapter 5). When wind velocity is high enough to slide or roll materials along the surface, the process is called creepA slow and steady movement. Used with faults, mass wasting in soils, and grain movement.">creep.
One extreme version of <span aria-describedby="tt" class="glossaryLink" data-cmtooltip="
">sediment movement was shrouded in mystery for years: <span aria-describedby="tt" class="glossaryLink" data-cmtooltip="
">Sliding stones. Also called <span aria-describedby="tt" class="glossaryLink" data-cmtooltip="
">sailing stones and <span aria-describedby="tt" class="glossaryLink" data-cmtooltip="
">sliding rocks, these are large moving boulders along flat surfaces in deserts, leaving trails. This includes the famous example of the Racetrack <span aria-describedby="tt" class="glossaryLink" data-cmtooltip="
">Playa in Death Valley National Park, California. For years, scientists and enthusiasts attempted to explain their movement, with little definitive results. In recent years, several experimental and observational studies have confirmed that the stones, imbedded in thin layers of ice, are propelled by friction from high winds. These studies include measurements of actual movement, as well as re-creations of the conditions, with resulting movement in the lab.
The zone of saltating sand is an effective agent of <span aria-describedby="tt" class="glossaryLink" data-cmtooltip="
">erosion through sand abrasion. A <span aria-describedby="tt" class="glossaryLink" data-cmtooltip="
">bedrock outcrop which has such a sandblasted shape is called a <span aria-describedby="tt" class="glossaryLink" data-cmtooltip="
">yardang. Rocks and boulders lying on the surface may be blasted and polished by saltating sand. When predominant wind directions shift, multiple sandblasted and polished faces may appear. Such wind abraded desert rocks are called <span aria-describedby="tt" class="glossaryLink" data-cmtooltip="
">ventifacts.
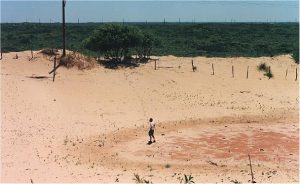
In places with sand and silt accumulations, clumps of vegetation often anchor <span aria-describedby="tt" class="glossaryLink" data-cmtooltip="
">sediment on the desert surface. Yet, winds may be sufficient to remove materials not anchored by vegetation. The bowl-shaped depression remaining on the surface is called a <span aria-describedby="tt" class="glossaryLink" data-cmtooltip="
">blowout.
13.3 Desert landforms
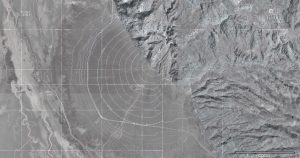
In the American Southwest, as <span aria-describedby="tt" class="glossaryLink" data-cmtooltip="
">streams emerge into the valleys from the adjacent mountains, they create desert landforms called <span aria-describedby="tt" class="glossaryLink" data-cmtooltip="
">alluvial fans. When the <span aria-describedby="tt" class="glossaryLink" data-cmtooltip="
">stream emerges from the narrow canyon, the flow is no longer constrained by the canyon walls and spreads out. At the lower slope angle, the water slows down and drops its coarser load. As the channel fills with this conglomeratic material, the <span aria-describedby="tt" class="glossaryLink" data-cmtooltip="
">stream is deflected around it. This deposited material deflects the <span aria-describedby="tt" class="glossaryLink" data-cmtooltip="
">stream into a <span aria-describedby="tt" class="glossaryLink" data-cmtooltip="
">system of radial distributary channels in a process similar to how a deltaPlace where rivers enter a large body of water, forming a triangular shape as the river deposits sediment and switches course.">delta is made by a <span aria-describedby="tt" class="glossaryLink" data-cmtooltip="
">river entering a body of water. This process develops a <span aria-describedby="tt" class="glossaryLink" data-cmtooltip="
">system of radial distributaries and constructs a fan shaped feature called an <span aria-describedby="tt" class="glossaryLink" data-cmtooltip="
">alluvial fan.
<span aria-describedby="tt" class="glossaryLink" data-cmtooltip="
">Alluvial fans continue to grow and may eventually coalesce with neighboring fans to form an apron of <span aria-describedby="tt" class="glossaryLink" data-cmtooltip="
">alluvium along the mountain front called a <span aria-describedby="tt" class="glossaryLink" data-cmtooltip="
">bajada.
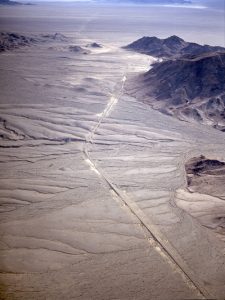
As the mountains erode away and their <span aria-describedby="tt" class="glossaryLink" data-cmtooltip="
">sediment accumulates first in <span aria-describedby="tt" class="glossaryLink" data-cmtooltip="
">alluvial fans, then <span aria-describedby="tt" class="glossaryLink" data-cmtooltip="
">bajadas, the mountains eventually are buried in their own erosional debris. Such buried mountain remnants are called <span aria-describedby="tt" class="glossaryLink" data-cmtooltip="
">inselbergs, “island mountains,” as first described by the German geologist Wilhelm Bornhardt (1864–1946).
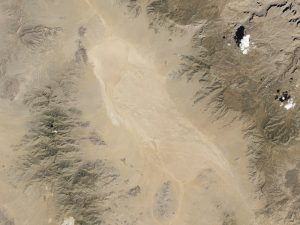
Where the desert valley is an enclosed <span aria-describedby="tt" class="glossaryLink" data-cmtooltip="
">basin, i.e. <span aria-describedby="tt" class="glossaryLink" data-cmtooltip="
">streams entering it do not drain out, the water is removed by evaporation and a dry lake bedA specific layer of rock with identifiable properties.">bed is formed called a <span aria-describedby="tt" class="glossaryLink" data-cmtooltip="
">playa.
<span aria-describedby="tt" class="glossaryLink" data-cmtooltip="
">Playas are among the flattest of all landforms. Such a dry lake bedA specific layer of rock with identifiable properties.">bed may cover a large area and be filled after a heavy thunderstorm to only a few inches deep. <span aria-describedby="tt" class="glossaryLink" data-cmtooltip="
">Playa lakes and desert <span aria-describedby="tt" class="glossaryLink" data-cmtooltip="
">streams that contain water only after rainstorms are called intermittent or <span aria-describedby="tt" class="glossaryLink" data-cmtooltip="
">ephemeral. Because of intense thunderstorms, the volume of water transported by <span aria-describedby="tt" class="glossaryLink" data-cmtooltip="
">ephemeral drainage in arid environments can be substantial during a short <span aria-describedby="tt" class="glossaryLink" data-cmtooltip="
">period of time. Desert soilA type of non-eroded sediment mixed with organic matter, used by plants. Many essential elements for life, like nitrogen, are delivered to organisms via the soil.">soil structures lack organic matter that promotes <span aria-describedby="tt" class="glossaryLink" data-cmtooltip="
">infiltration by absorbing water. Instead of percolating into the soilA type of non-eroded sediment mixed with organic matter, used by plants. Many essential elements for life, like nitrogen, are delivered to organisms via the soil.">soil, the <span aria-describedby="tt" class="glossaryLink" data-cmtooltip="
">runoff compacts the ground surface, making the soilA type of non-eroded sediment mixed with organic matter, used by plants. Many essential elements for life, like nitrogen, are delivered to organisms via the soil.">soil hydrophobic or water-repellant. Because of this hardpan surface, <span aria-describedby="tt" class="glossaryLink" data-cmtooltip="
">ephemeral <span aria-describedby="tt" class="glossaryLink" data-cmtooltip="
">streams may gather water across large areas, suddenly filling with water from storms many miles away.
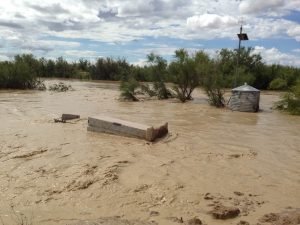
High-volume <span aria-describedby="tt" class="glossaryLink" data-cmtooltip="
">ephemeral flows, called <span aria-describedby="tt" class="glossaryLink" data-cmtooltip="
">flash floods, may move as sheet flows or <span aria-describedby="tt" class="glossaryLink" data-cmtooltip="
">sheetwash, as well as be channeled through normally dry <span aria-describedby="tt" class="glossaryLink" data-cmtooltip="
">arroyos or canyons. Flash floods are a major factor in desert <span aria-describedby="tt" class="glossaryLink" data-cmtooltip="
">deposition. Dry channels can fill quickly with <span aria-describedby="tt" class="glossaryLink" data-cmtooltip="
">ephemeral <span aria-describedby="tt" class="glossaryLink" data-cmtooltip="
">drainage, creating a mass of water and debris that charges down the <span aria-describedby="tt" class="glossaryLink" data-cmtooltip="
">arroyo, and even overflowing the banks. <span aria-describedby="tt" class="glossaryLink" data-cmtooltip="
">Flash floods pose a serious hazard for desert travelers because the storm activity feeding the <span aria-describedby="tt" class="glossaryLink" data-cmtooltip="
">runoff may be miles away. People hiking or camping in <span aria-describedby="tt" class="glossaryLink" data-cmtooltip="
">arroyos that have been bone dry for months, or years, have been swept away by sudden <span aria-describedby="tt" class="glossaryLink" data-cmtooltip="
">flash floods.
13.3.1 Sand
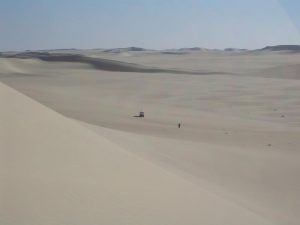
The popular concept of a typical desert is a broad expanse of sand. Geologically, deserts are defined by a lack of water and arid regions resembling a sea of sand belong to the category of desert called an ergA vast stretch of sand dunes.">erg. An ergA vast stretch of sand dunes.">erg consists of fine-grained, loose sand grains, often blown by wind, or <span aria-describedby="tt" class="glossaryLink" data-cmtooltip="
">aeolian forces, into duneA large pile of sediment, deposited perpendicular to flow. Internal bedding in dunes dips toward flow direction (i.e. cross bedding). Formed in the upper part of the lower flow regime.">dunes. Probably the best known ergA vast stretch of sand dunes.">erg is the Rub’ al Khali, which means Empty Quarter, of the Arabian Peninsula. Ergs are also found in the Great Sand duneA large pile of sediment, deposited perpendicular to flow. Internal bedding in dunes dips toward flow direction (i.e. cross bedding). Formed in the upper part of the lower flow regime.">Dunes National Park (Colorado), Little Sahara Recreation Area (Utah), White Sands National Monument (New Mexico), and parts of Death Valley National Park (California). ergA vast stretch of sand dunes.">Ergs are not restricted to deserts, but may form anywhere there is a substantial supply of sand, including as far north as 60° N in Saskatchewan, Canada, in the Athabasca Sand duneA large pile of sediment, deposited perpendicular to flow. Internal bedding in dunes dips toward flow direction (i.e. cross bedding). Formed in the upper part of the lower flow regime.">Dunes Provincial Park . Coastal ergA vast stretch of sand dunes.">ergs exist along lakes and oceans as well, and examples are found in Oregon, Michigan, and Indiana.
An internal cross section of a sand duneA large pile of sediment, deposited perpendicular to flow. Internal bedding in dunes dips toward flow direction (i.e. cross bedding). Formed in the upper part of the lower flow regime.">dune shows a feature called <span aria-describedby="tt" class="glossaryLink" data-cmtooltip="
">cross bedding. As wind blows up the windward side of the duneA large pile of sediment, deposited perpendicular to flow. Internal bedding in dunes dips toward flow direction (i.e. cross bedding). Formed in the upper part of the lower flow regime.">dune, it carries sand to the duneA large pile of sediment, deposited perpendicular to flow. Internal bedding in dunes dips toward flow direction (i.e. cross bedding). Formed in the upper part of the lower flow regime.">dune crest depositing layers of sand parallel to the windward (or “stoss”) side. The sand builds up the crest of the duneA large pile of sediment, deposited perpendicular to flow. Internal bedding in dunes dips toward flow direction (i.e. cross bedding). Formed in the upper part of the lower flow regime.">dune and pours over the top until the leeward (downwind or slip) face of the duneA large pile of sediment, deposited perpendicular to flow. Internal bedding in dunes dips toward flow direction (i.e. cross bedding). Formed in the upper part of the lower flow regime.">dune reaches the <span aria-describedby="tt" class="glossaryLink" data-cmtooltip="
">angle of repose, the maximum angle which will support the slip face. duneA large pile of sediment, deposited perpendicular to flow. Internal bedding in dunes dips toward flow direction (i.e. cross bedding). Formed in the upper part of the lower flow regime.">Dunes are unstable features and move as the sand erodes from the stoss side and continues to drop down the leeward side covering previous stoss and slip-face layers and creating the cross bedA specific layer of rock with identifiable properties.">beds. Mostly, these are reworked over and over again, but occasionally, the features are preserved in a depression, then lithified. Shifting wind directions and abundant sand sources create chaotic patterns of cross bedA specific layer of rock with identifiable properties.">beds like those seen in Zion National Park of Utah.
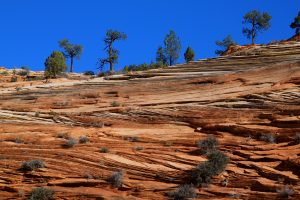
In the <span aria-describedby="tt" class="glossaryLink" data-cmtooltip="
">Mesozoic eraThe second largest span of time recognized by geologists; smaller than a eon, larger than a period. We are currently in the Cenozoic era. Rocks of a specific era are called eratherms.">Era, Utah was covered by a series of ergA vast stretch of sand dunes.">ergs, with the thickest being in Southern Utah, which lithified into <span aria-describedby="tt" class="glossaryLink" data-cmtooltip="
">sandstone (see Chapter 5). Perhaps the best known of these <span aria-describedby="tt" class="glossaryLink" data-cmtooltip="
">sandstone <span aria-describedby="tt" class="glossaryLink" data-cmtooltip="
">formations is the Navajo <span aria-describedby="tt" class="glossaryLink" data-cmtooltip="
">Sandstone of <span aria-describedby="tt" class="glossaryLink" data-cmtooltip="
">Jurassic age. This <span aria-describedby="tt" class="glossaryLink" data-cmtooltip="
">sandstone formation consists of dramatic cliffs and spires in Zion National Park and covers a large part of the Colorado Plateau. In Arches National Park, a later series of sand duneA large pile of sediment, deposited perpendicular to flow. Internal bedding in dunes dips toward flow direction (i.e. cross bedding). Formed in the upper part of the lower flow regime.">dunes covered the Navajo <span aria-describedby="tt" class="glossaryLink" data-cmtooltip="
">Sandstone and lithified to become the Entrada <span aria-describedby="tt" class="glossaryLink" data-cmtooltip="
">Formation also during the <span aria-describedby="tt" class="glossaryLink" data-cmtooltip="
">Jurassic. <span aria-describedby="tt" class="glossaryLink" data-cmtooltip="
">Erosion of overlying layers exposed fins of the underlying Entrada <span aria-describedby="tt" class="glossaryLink" data-cmtooltip="
">Sandstone and carved out weaker parts of the fins forming the arches.
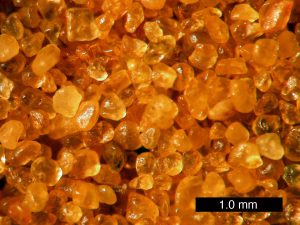
As the cements that hold the grains together in these modern sand cliffs disintegrate and the freed grains gather at the base of the cliffs and move down the washes, sand grains may be recycled and redeposited. These <span aria-describedby="tt" class="glossaryLink" data-cmtooltip="
">Mesozoic sand ergA vast stretch of sand dunes.">ergs may represent ancient <span aria-describedby="tt" class="glossaryLink" data-cmtooltip="
">quartz sands recycled many times from <span aria-describedby="tt" class="glossaryLink" data-cmtooltip="
">igneous origins in the early <span aria-describedby="tt" class="glossaryLink" data-cmtooltip="
">Precambrian, just passing now through another cycle of <span aria-describedby="tt" class="glossaryLink" data-cmtooltip="
">erosion and <span aria-describedby="tt" class="glossaryLink" data-cmtooltip="
">deposition. An example of this is Coral Pink Sand duneA large pile of sediment, deposited perpendicular to flow. Internal bedding in dunes dips toward flow direction (i.e. cross bedding). Formed in the upper part of the lower flow regime.">Dunes State Park in Southwestern Utah, which contains sand that is being eroded from the Navajo <span aria-describedby="tt" class="glossaryLink" data-cmtooltip="
">Sandstone to form new duneA large pile of sediment, deposited perpendicular to flow. Internal bedding in dunes dips toward flow direction (i.e. cross bedding). Formed in the upper part of the lower flow regime.">dunes.
Dune Types
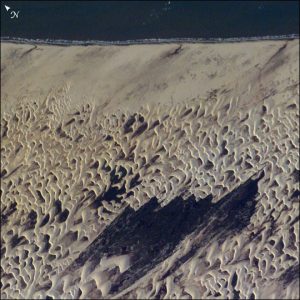
duneA large pile of sediment, deposited perpendicular to flow. Internal bedding in dunes dips toward flow direction (i.e. cross bedding). Formed in the upper part of the lower flow regime.">Dunes are complex features formed by a combination of wind direction and sand supply, in some cases interacting with vegetation. There are several types of duneA large pile of sediment, deposited perpendicular to flow. Internal bedding in dunes dips toward flow direction (i.e. cross bedding). Formed in the upper part of the lower flow regime.">dunes representing variables of wind direction, sand supply and vegetative anchoring. Barchan duneA large pile of sediment, deposited perpendicular to flow. Internal bedding in dunes dips toward flow direction (i.e. cross bedding). Formed in the upper part of the lower flow regime.">dunes or crescent duneA large pile of sediment, deposited perpendicular to flow. Internal bedding in dunes dips toward flow direction (i.e. cross bedding). Formed in the upper part of the lower flow regime.">dunes form where sand supply is limited and there is a fairly constant wind direction. Barchans move downwind and develop a crescent shape with wings on either side of a duneA large pile of sediment, deposited perpendicular to flow. Internal bedding in dunes dips toward flow direction (i.e. cross bedding). Formed in the upper part of the lower flow regime.">dune crest. Barchans are known to actually move over homes, even towns.

<span aria-describedby="tt" class="glossaryLink" data-cmtooltip="
">Longitudinal dunes or <span aria-describedby="tt" class="glossaryLink" data-cmtooltip="
">linear dunes form where sand supply is greater and the wind blows around a dominant direction, in a back-and-forth manner. They may form ridges tens of meters high lined up with the predominant wind directions.
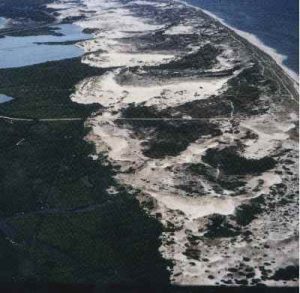
<span aria-describedby="tt" class="glossaryLink" data-cmtooltip="
">Parabolic dunes form where vegetation anchors parts of the sand and unanchored parts <span aria-describedby="tt" class="glossaryLink" data-cmtooltip="
">blowout. <span aria-describedby="tt" class="glossaryLink" data-cmtooltip="
">Parabolic dune shape may be similar to barchan duneA large pile of sediment, deposited perpendicular to flow. Internal bedding in dunes dips toward flow direction (i.e. cross bedding). Formed in the upper part of the lower flow regime.">dunes but usually reversed, and it is determined more by the anchoring vegetation than a strict parabolic form.
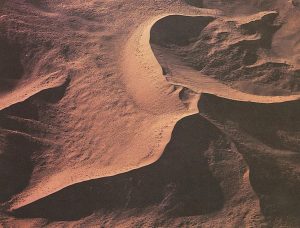
<span aria-describedby="tt" class="glossaryLink" data-cmtooltip="
">Star dunes form where the wind direction is variable in all directions. Sand supply can range from limited to quite abundant. It is the variation in wind direction that forms the star.
13.4 The Great Basin and the Basin and Range
The Great <span aria-describedby="tt" class="glossaryLink" data-cmtooltip="
">Basin is the largest area of interior <span aria-describedby="tt" class="glossaryLink" data-cmtooltip="
">drainage in North America, meaning there is no outlet to the ocean and all <span aria-describedby="tt" class="glossaryLink" data-cmtooltip="
">precipitation remains in the <span aria-describedby="tt" class="glossaryLink" data-cmtooltip="
">basin or is evaporated. It covers western Utah, most of Nevada, and extends into southeastern California, southern Oregon, and southern Idaho. Because there is not outlet to the ocean, <span aria-describedby="tt" class="glossaryLink" data-cmtooltip="
">streams in the Great <span aria-describedby="tt" class="glossaryLink" data-cmtooltip="
">Basin deliver <span aria-describedby="tt" class="glossaryLink" data-cmtooltip="
">runoff to lakes and <span aria-describedby="tt" class="glossaryLink" data-cmtooltip="
">playas within the <span aria-describedby="tt" class="glossaryLink" data-cmtooltip="
">basin. A subregion within the Great <span aria-describedby="tt" class="glossaryLink" data-cmtooltip="
">Basin is the <span aria-describedby="tt" class="glossaryLink" data-cmtooltip="
">Basin and Range which extends from the Wasatch Front in Utah west across Nevada to the Sierra Nevada Mountains of California. The basins and ranges referred to in the name are <span aria-describedby="tt" class="glossaryLink" data-cmtooltip="
">horsts and <span aria-describedby="tt" class="glossaryLink" data-cmtooltip="
">grabens, formed by <span aria-describedby="tt" class="glossaryLink" data-cmtooltip="
">normal fault blocks from crustal <span aria-describedby="tt" class="glossaryLink" data-cmtooltip="
">extension, as discussed in chapter 2 and chapter 9. The <span aria-describedby="tt" class="glossaryLink" data-cmtooltip="
">lithosphere of the entire area has stretched by a factor of about 2, meaning from end to end, the distance has doubled over the past 30 million years or so. Valleys without outlets form individual basins, each of which is filled with <span aria-describedby="tt" class="glossaryLink" data-cmtooltip="
">alluvial <span aria-describedby="tt" class="glossaryLink" data-cmtooltip="
">sediments leading into <span aria-describedby="tt" class="glossaryLink" data-cmtooltip="
">playa <span aria-describedby="tt" class="glossaryLink" data-cmtooltip="
">depositional environments. During the recent <span aria-describedby="tt" class="glossaryLink" data-cmtooltip="
">Ice Age, The <span aria-describedby="tt" class="glossaryLink" data-cmtooltip="
">climate was more humid and while <span aria-describedby="tt" class="glossaryLink" data-cmtooltip="
">glaciers were forming in some of the mountains, <span aria-describedby="tt" class="glossaryLink" data-cmtooltip="
">pluvial lakes formed covering large areas (see chapter 14.4.3). Valleys in much of western Utah and eastern Nevada were covered by Lake Bonneville. As the <span aria-describedby="tt" class="glossaryLink" data-cmtooltip="
">climate became arid after the <span aria-describedby="tt" class="glossaryLink" data-cmtooltip="
">Ice Age, Lake Bonneville dried leaving as a remnant the Great Salt Lake in Utah.
The desert of the <span aria-describedby="tt" class="glossaryLink" data-cmtooltip="
">Basin and Range extends from about 35° to near 40° and results from a rain shadow effect created by westerly winds from the Pacific rising and cooling over the Sierras becoming depleted of moisture by <span aria-describedby="tt" class="glossaryLink" data-cmtooltip="
">precipitation on the western side. The result is relatively dry air descending across Nevada and western Utah.
A journey from the Wasatch Front southwest to the Pacific Ocean will show stages of desert landscape evolution from the <span aria-describedby="tt" class="glossaryLink" data-cmtooltip="
">fault block mountains of Utah with sharp peaks and <span aria-describedby="tt" class="glossaryLink" data-cmtooltip="
">alluvial fans at the mouths of canyons, through landscapes in Southern Nevada with <span aria-describedby="tt" class="glossaryLink" data-cmtooltip="
">bajadas along the mountain fronts, to the landscapes in the Mojave Desert of California with subdued <span aria-describedby="tt" class="glossaryLink" data-cmtooltip="
">inselbergs sticking up through a sea of coalesced <span aria-describedby="tt" class="glossaryLink" data-cmtooltip="
">bajadas. These landscapes illustrate the evolutionary stages of desert landscape development.
13.4.1 Desertification
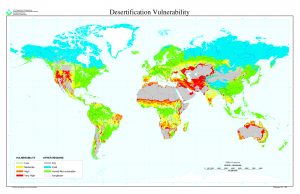
When previously arable land suitable for agriculture transforms into desert, this process is called <span aria-describedby="tt" class="glossaryLink" data-cmtooltip="
">desertification. Plants and <span aria-describedby="tt" class="glossaryLink" data-cmtooltip="
">humus-rich soilA type of non-eroded sediment mixed with organic matter, used by plants. Many essential elements for life, like nitrogen, are delivered to organisms via the soil.">soil (see Chapter 5) promote <span aria-describedby="tt" class="glossaryLink" data-cmtooltip="
">groundwater <span aria-describedby="tt" class="glossaryLink" data-cmtooltip="
">infiltration and water retention. When an area becomes more arid due to changing environmental conditions, the plants and soilA type of non-eroded sediment mixed with organic matter, used by plants. Many essential elements for life, like nitrogen, are delivered to organisms via the soil.">soil become less effective in retaining water, creating a <span aria-describedby="tt" class="glossaryLink" data-cmtooltip="
">positive feedback loop of <span aria-describedby="tt" class="glossaryLink" data-cmtooltip="
">desertification. This self-reinforcing loop spirals into increasingly arid conditions and further enlarges the desert regions.
<span aria-describedby="tt" class="glossaryLink" data-cmtooltip="
">Desertification may be caused by human activities, such as unsustainable crop cultivation practices, overgrazing by livestock, overuse of groundwater, and global <span aria-describedby="tt" class="glossaryLink" data-cmtooltip="
">climate change. Human-caused desertification is a serious worldwide problem. The world map figure above shows what areas are most vulnerable to <span aria-describedby="tt" class="glossaryLink" data-cmtooltip="
">desertification. Note the red and orange areas in the western and midwestern regions of the United States, which also cover large areas of arable land used for raising food crops and animals. The creation of the Dust Bowl in the 1930s (see Chapter 5) is a classic example of a high-vulnerability region impacted by human–caused <span aria-describedby="tt" class="glossaryLink" data-cmtooltip="
">desertification. As demonstrated in the Dust Bowl, conflicts may arise between agricultural practices and conservation measures. Mitigating <span aria-describedby="tt" class="glossaryLink" data-cmtooltip="
">desertification while allowing farmers to make a survivable living requires public and individual education to create community support and understanding of sustainable agriculture alternatives.
Summary
Approximately 30% of Earth’s surface is arid lands, the location of which is determined by <span aria-describedby="tt" class="glossaryLink" data-cmtooltip="
">latitude, atmospheric circulation, and terrain. The arid belts between 15o and 30o north and south latitudes are produced by descending air masses associated with major cells in the <span aria-describedby="tt" class="glossaryLink" data-cmtooltip="
">atmosphere and include the major deserts like the Sahara in Africa and the Middle East. <span aria-describedby="tt" class="glossaryLink" data-cmtooltip="
">Rain shadow deserts lie behind mountain ranges or long land expanses in zones of prevailing winds like the deserts of western North America, the Atacama of South America, and the Gobi of Asia. Dry descending air also creates the <span aria-describedby="tt" class="glossaryLink" data-cmtooltip="
">polar deserts at the poles.
Major atmospheric circulation involves the <span aria-describedby="tt" class="glossaryLink" data-cmtooltip="
">Hadley cells, midlatitude or Ferrel cells, and the <span aria-describedby="tt" class="glossaryLink" data-cmtooltip="
">polar cells in each hemisphere. Warmed and rising air in the <span aria-describedby="tt" class="glossaryLink" data-cmtooltip="
">Hadley cells rains back on the tropics and moves toward the poles as dryer air. That air meets the dryer equatorward moving air of the Ferrel cells. This dry air descends in the arid zones, called the horse latitudes, to produce the arid belts in each hemisphere. Rotation of the Earth creates the Coriolis Effect that deflects these moving air masses to produce zones of prevailing winds, the <span aria-describedby="tt" class="glossaryLink" data-cmtooltip="
">Trade Winds in the subtropics and the <span aria-describedby="tt" class="glossaryLink" data-cmtooltip="
">Westerlies in the midlatitudes. A combination of <span aria-describedby="tt" class="glossaryLink" data-cmtooltip="
">latitude, rain shadow, and cold adjacent ocean currents causes the <span aria-describedby="tt" class="glossaryLink" data-cmtooltip="
">Atacama Desert of northern Chile, the driest desert on Earth.
<span aria-describedby="tt" class="glossaryLink" data-cmtooltip="
">Weathering in deserts takes place just as in other climes only slower because of less water. <span aria-describedby="tt" class="glossaryLink" data-cmtooltip="
">Desert varnish is a <span aria-describedby="tt" class="glossaryLink" data-cmtooltip="
">weathering product unique to desert environments. As in more humid climes, water is the main agent of <span aria-describedby="tt" class="glossaryLink" data-cmtooltip="
">erosion although wind is a notable agent. Large dust storms called <span aria-describedby="tt" class="glossaryLink" data-cmtooltip="
">haboobs transport large amounts of <span aria-describedby="tt" class="glossaryLink" data-cmtooltip="
">sediment that may accumulate in sand seas called ergA vast stretch of sand dunes.">ergs or finer grained <span aria-describedby="tt" class="glossaryLink" data-cmtooltip="
">loess deposits. Sand transport occurs mainly by <span aria-describedby="tt" class="glossaryLink" data-cmtooltip="
">saltation in which grain to grain impact causes frosting of grain surfaces. Sandblasting by persistent winds produces stones with polished surfaces called <span aria-describedby="tt" class="glossaryLink" data-cmtooltip="
">ventifacts and sculpted <span aria-describedby="tt" class="glossaryLink" data-cmtooltip="
">bedrock features called <span aria-describedby="tt" class="glossaryLink" data-cmtooltip="
">yardangs.
Landforms produced in desert environments include <span aria-describedby="tt" class="glossaryLink" data-cmtooltip="
">alluvial fans, <span aria-describedby="tt" class="glossaryLink" data-cmtooltip="
">bajadas, <span aria-describedby="tt" class="glossaryLink" data-cmtooltip="
">inselbergs, and <span aria-describedby="tt" class="glossaryLink" data-cmtooltip="
">playas. Windblown sand can accumulate as duneA large pile of sediment, deposited perpendicular to flow. Internal bedding in dunes dips toward flow direction (i.e. cross bedding). Formed in the upper part of the lower flow regime.">dunes. The forms of duneA large pile of sediment, deposited perpendicular to flow. Internal bedding in dunes dips toward flow direction (i.e. cross bedding). Formed in the upper part of the lower flow regime.">dunes, like barchans, parabolic, longitudinal, and start duneA large pile of sediment, deposited perpendicular to flow. Internal bedding in dunes dips toward flow direction (i.e. cross bedding). Formed in the upper part of the lower flow regime.">dunes, relate to the abundance of sand supply and wind direction as well as presence of vegetation. The internal structure of duneA large pile of sediment, deposited perpendicular to flow. Internal bedding in dunes dips toward flow direction (i.e. cross bedding). Formed in the upper part of the lower flow regime.">dunes shows <span aria-describedby="tt" class="glossaryLink" data-cmtooltip="
">cross bedding. <span aria-describedby="tt" class="glossaryLink" data-cmtooltip="
">Fossil duneA large pile of sediment, deposited perpendicular to flow. Internal bedding in dunes dips toward flow direction (i.e. cross bedding). Formed in the upper part of the lower flow regime.">dunes in an ancient desert leave <span aria-describedby="tt" class="glossaryLink" data-cmtooltip="
">cross bedding in places like Zion and Arches national parks in Utah showing shifting wind directions in these ancient environments. <span aria-describedby="tt" class="glossaryLink" data-cmtooltip="
">Ephemeral <span aria-describedby="tt" class="glossaryLink" data-cmtooltip="
">streams in desert regions may carry water only after storms and pose risk of <span aria-describedby="tt" class="glossaryLink" data-cmtooltip="
">flash floods.
The Great <span aria-describedby="tt" class="glossaryLink" data-cmtooltip="
">Basin of North America is an enclosed <span aria-describedby="tt" class="glossaryLink" data-cmtooltip="
">basin with no <span aria-describedby="tt" class="glossaryLink" data-cmtooltip="
">drainage to the ocean. The only exit for <span aria-describedby="tt" class="glossaryLink" data-cmtooltip="
">precipitation there is by evaporation. Travels in the Great <span aria-describedby="tt" class="glossaryLink" data-cmtooltip="
">Basin show stages of development of desert landscapes from <span aria-describedby="tt" class="glossaryLink" data-cmtooltip="
">playas and <span aria-describedby="tt" class="glossaryLink" data-cmtooltip="
">alluvial fans, to <span aria-describedby="tt" class="glossaryLink" data-cmtooltip="
">bajadas, to <span aria-describedby="tt" class="glossaryLink" data-cmtooltip="
">inselbergs which are eroded mountains buried in their own erosional debris.
Poor land management can result in dying vegetation and loss of soilA type of non-eroded sediment mixed with organic matter, used by plants. Many essential elements for life, like nitrogen, are delivered to organisms via the soil.">soil moisture producing an accelerating process of <span aria-describedby="tt" class="glossaryLink" data-cmtooltip="
">desertification in which once productive land is degraded into unproductive desert. This is a serious worldwide problem.
References
Scientific Method in Geosciences: Multiple hypotheses, Multiple modes of Inquiry
How is science made in Earth Sciences? Why should you care? Even if you are not interested in becoming an Earth scientist, or any kind of scientist, understanding how science works is a source of empowerment. This is because science and scientific thinking are at the heart of our modern way of life and they influence every aspect of our lives. Understanding how science works can help you discern fact from fiction and inform your life choices and political decisions.
Modern science is based on the scientific method, the idea in science that phenomena and ideas need to be scrutinized using hypothesizing, experimentation, and analysis. This can eventually result in a consensus or scientific theory.
The scientific method, is a procedure that follows these steps:
- Formulate a question or observe a problem
- Apply objective experimentation and observation
- Analyze collected data and Interpret results.
- Devise an evidence-based theory.
- Submit findings to peer review.
Let's see how scientific inquiry works in Earth science.
1. Observation, problem, or research question
The procedure begins when scientists identify a problem or research question, such as a geological phenomenon that is not well explained in the scientific community’s collective knowledge. This step usually involves reviewing the scientific literature to establish what is known and to consult previous studies related to the question.
Earth scientists can study processes they cannot observe directly, because they happen over long periods of time, or happened long ago, or happen in a remote location. An example of the last one would be the Earth's core. To circumvent these challenges, Earth scientists have developed strategies to test their hypothesis, these strategies make up the scientific method of geosciences.
2. Hypothesis
Once the scientists define the problem or question, they propose a possible answer, a hypothesis
hypotheses to gather data from multiple lines. To test hypotheses, scientists use methods drawn from other sciences such as chemistry, physics, biology, or even engineering.
3. Testing Hypotheses: Experiments and Revisions

The next step is developing an <span aria-describedby="tt" data-cmtooltip="
Earth scientists conduct classic experiments in the lab, however, an
An experiment can take other forms such as:
- Observing natural processes and their products in the field and comparing them to those found in the rock record. E.g., A sedimentologist studies how wind moves and forms dunes and different ripples in a desert. This knowledge helps her to interpret ripple structures found in rocks, and even to interpret dunes and aeolian processes from images collected on Mars!
- Studying changes across time or space. E.g., an atmospheric scientist analyzes how the composition of the atmosphere has changed since we started measuring it.
- Using physical models. This is more akin to the "classic experiment". E.g., a team of scientists build a model for landslides using a long and steep ramp and flushing down different materials, and changing the ramp angle.
- Using computer models. E.g., climatologists develop computational models to study the climate system and make predictions. These scientific models undergo rigorous scrutiny and testing by collaborating and competing groups of scientists around the world.
- Considering multiple lines of evidence. To establish a scientific finding, all lines of evidence must converge, that means that all the results you collect using different methods must agree with the finding, the math must be sound, and the methods must be thoroughly described.
Regardless of what form an <span aria-describedby="tt" data-cmtooltip="
">experiment takes, it always includes the systematic gathering of <span aria-describedby="tt" data-cmtooltip="
">objective data. The scientists interpret this data to determine whether it contradicts or supports the <span aria-describedby="tt" data-cmtooltip="
">hypothesis (step 2). If the results contradict the hypothesis, then scientists can revise it and test it again. When a <span aria-describedby="tt" data-cmtooltip="
">hypothesis holds up under experimentation, it is ready to be shared with other experts in the field. The findings are scrutinized by the scientific community through the process of peer review.
Backyard Geology: Arizona Wildfire Study in Saguaro National Park
Arizona ranks high in the number of individual fires, as well as acres burned, every year. Below are the top 10 states for wildfires:
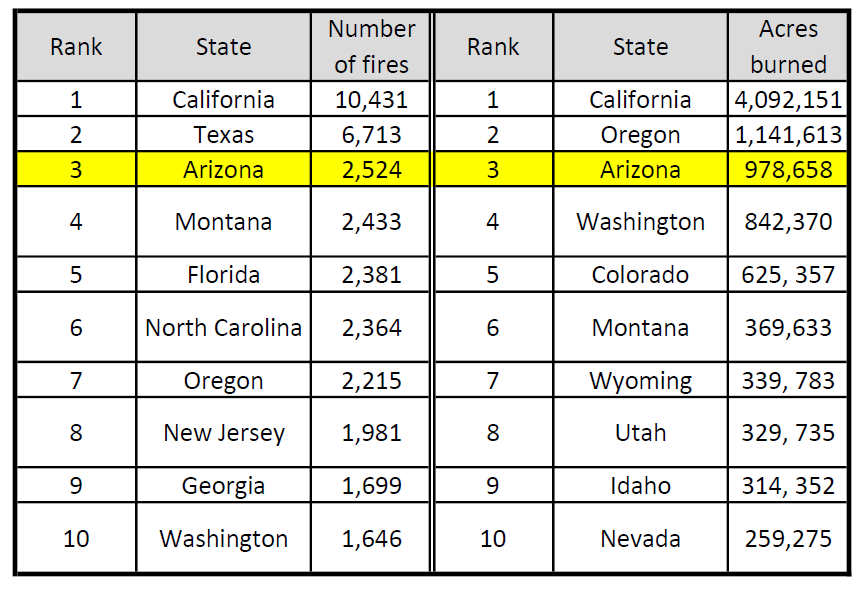
In 2020, Arizona ranked 3rd out of all states in both categories, with 2,524 individual fires and 978,568 acres burned. Why does Arizona have so many fires? Wildfire risk depends on many factors, including temperature, soil moisture, and the presence of vegetation (fuel) to burn. Certainly the dry, hot climate plays a role, and climate change intensifies drought conditions, but what contributes to the fuel available to burn?
It was determined in a previous study that invasive buffelgrass is fire-adapted, meaning it establishes, persists, and spreads following fire. It also outcompetes native vegetation for resources, supplies material that can be readily burned, and connects areas subjected to wildfires. Therefore, can we control or remove buffelgrass in the Arizona wilderness?
- Research Question: Invasive buffelgrass contributes to wildfires, can it be controlled or removed in wilderness areas?
- Hypothesis: If buffelgrass can be controlled, the intensity and interconnectedness of wildfires will lessen and cause less devastation.
- Experiment: Herbicides were used to control dense patches of buffelgrass in remote areas. The herbicide was applied with the use of helicopters.
- Results:
4. Peer review, publication, and replication
Science is a social process. Scientists share the results of their research in conferences and by publishing articles in scientific journals, such as Science and Nature. Reputable journals and academic outlets will not publish an experimental study until they have determined its methods are scientifically rigorous and the evidence supports the conclusions. Before they publish the article, scientific experts in the field scrutinize the methods, results, and discussion; the peer review process. Once an article is published, other scientists may attempt to replicate the results. This replication is necessary to confirm the reliability of the study’s reported results. A <span aria-describedby="tt" data-cmtooltip="
">hypothesis that seemed compelling in one study might be proven false in studies conducted by other scientists. New technology can be applied to published studies, which can aid in confirming or rejecting once-accepted ideas and/or <span aria-describedby="tt" data-cmtooltip="
">hypotheses.
5. Theory development

In casual conversation, the word <span aria-describedby="tt" data-cmtooltip="
">theory implies guesswork or speculation. In the language of science, an explanation or conclusion made into a <span aria-describedby="tt" data-cmtooltip="
">theory carries much more weight because experiments support it and the scientific community widely accepts it. A <span aria-describedby="tt" data-cmtooltip="
">hypothesis that has been repeatedly confirmed through documented and independent studies eventually becomes accepted as a scientific <span aria-describedby="tt" data-cmtooltip="
">theory.
While a <span aria-describedby="tt" data-cmtooltip="
">hypothesis provides a tentative explanation before an <span aria-describedby="tt" data-cmtooltip="
">experiment, a <span aria-describedby="tt" data-cmtooltip="
">theory is the best explanation after being confirmed by multiple independent experiments. Confirmation of a <span aria-describedby="tt" data-cmtooltip="
">theory may take years, or even longer. For example, the scientific community initially dismissed the <span aria-describedby="tt" data-cmtooltip="
">continental drift <span aria-describedby="tt" data-cmtooltip="
">hypothesis first proposed by Alfred Wegener in 1912 (see Chapter 2). After decades of additional evidence collection by other scientists using more advanced technology, Wegener’s <span aria-describedby="tt" data-cmtooltip="
">hypothesis was accepted and revised as the <span aria-describedby="tt" data-cmtooltip="
">theory of <span aria-describedby="tt" data-cmtooltip="
The <span aria-describedby="tt" data-cmtooltip="
">theory of evolution by natural selection is another example. Originating from the work of Charles Darwin in the mid-19th century, the <span aria-describedby="tt" data-cmtooltip="
">theory of evolution has withstood generations of scientific testing for falsifiability. It has been updated and revised to accommodate knowledge gained by using modern technologies, but the latest evidence continues to support the <span aria-describedby="tt" data-cmtooltip="
">theory of evolution.
Science
We have covered the scientific method of the geosciences. However, science is a human endeavor. For generations, Indigenous peoples have accrued empirical knowledge of the natural world, including the lithosphere. Greg Cajete uses the name Native Science to refer to "the collective heritage of human experience with the natural world" (Cajete, 2000). Geologic expertise served tribal peoples in many of the same ways that Western geology serves modern civilizations. For example, Native Americans in Cascadia record volcanic events in stories, this transmits cross-generational awareness of volcanic hazards; the Muiscas in Colombia knew how to find, mine and process the gold to make beautiful art pieces; and the Puebloans of the North American Southwest managing limited water resources using an impressive net of canals that we still use for our benefit. Western and Native scientists can collaborate to further our understanding of the Earth systems and to remember how to live well on our planet.
Science is a dynamic process. Technological advances, breakthroughs in interpretation, and new observations continuously refine our understanding of Earth. We will never stop learning about our Earth. As new findings are published, we must revise and update our scientific knowledge and discard ideas that are proven false by new observations. Science is a living entity.
In conclusion, Earth scientists do not use a single, all-encompassing "scientific method". Instead, multiple modes of inquiry respond to the complexity and spatial and temporal scales of Earth systems.
"The unique thing about the geosciences is that the knowledge, skills, and methods are brought together, refined, and evolved over time to make them most suitable for understanding the complex processes of Earth, its working in the past and the present, and its likely behavior in the future." (Manduca and Kastens, 2012).
Key Takeaways
- Earth scientists work on challenging problems that face humanity on topics such as climate change, human impacts on Earth, and hazards to humans.
- Besides the classical laboratory experiment, Earth scientists construct models or use indirect methods to study the Earth.
- Scientific results are not valid or useful unless other scientists can reproduce them. Research results undergo scrutiny by the community before and after being published.
- The study of geological and environmental issues requires multiple disciplines and the interplay of multiple methods.
- Scientific thinking advances through collaboration and community.
GeoEthics

Geoscientists must act in ethical ways to contribute to the welfare of human beings. The saying "with great knowledge comes great responsibility" holds true for Earth and environmental scientists. Earth scientist must uphold high standards in research and conduct. The research and reflection upon the values which underpin behaviors and practices between humans and Earth systems is the arena of the "Geoethics" (Di Capua and Peppoloni, 2019). The International Association for Promoting Geoethics (IAPG) provide tools to "understand the complex relationship between human action on ecosystems and the decisions geoscientists make in the discipline that impact society, including improving the awareness of professionals, students, decision-makers, media operators, and the public on an accountable and ecologically sustainable development." Source: https://www.geoethics.org/geoethics-school
All of us, human beings, have responsibilities to Earth. We do not exist apart from this planet and our behaviors impact other people, other species, the larger biosphere, hydrosphere, atmosphere, and lithosphere. Our actions impact the Earth system. As you progress in your learning, strive to think critically and to identify ethical issues. Do not be afraid to question and discuss your observations with your instructor and with peers. Just remember to do so respectfully, considering other points of view and practicing active listening.
Gases dissolved within magma or lava.
molten rock that has erupted at the Earth's surface due to volcanic processes.