5.5 Where are the Volcanoes?
Distribution of Volcanic Activity
There are between 1400 and 1500 active volcanoes on our planet right now, but they are not evenly distributed throughout Earth’s surface. Some volcanoes cluster in specific regions. One infamous area that loosely spans the border between continents and the Pacific Ocean is known as the Ring of Fire. This region spans about 40,000 kilometers and it has significantly more volcanic and seismic activity compared with other places on the planet.
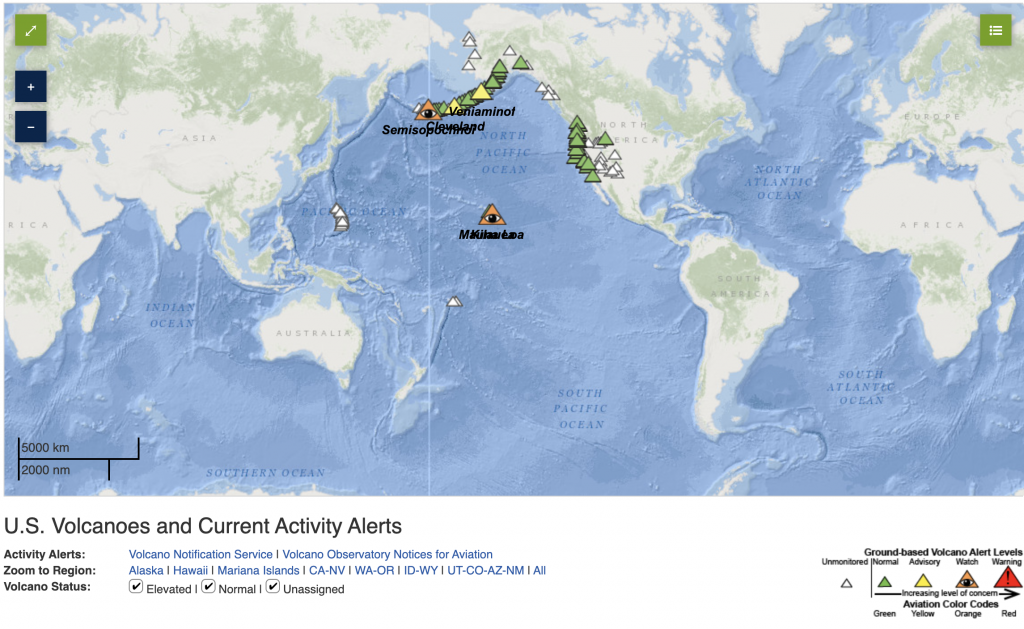
Why are so many volcanoes bunched together? Most volcanoes are formed by tectonic processes such as subduction at convergent boundaries or rifting at divergent boundaries. Therefore, the distribution of volcanoes naturally coincides with plate boundaries. This connection between volcanism and plate boundaries is called interplate volcanism. There are some volcanoes, however, that occur very far away from plate boundaries. They are typically the result of hot spot volcanism, which can occur on both continental and oceanic lithosphere.
Below we will explore some of the broad geologic environments that result in volcanism and the types of volcanoes we might expect from each.
Mid-Ocean Ridges
New lithosphere is continuously being formed at divergent boundaries. As two tectonic plates spread apart, the magma from the asthenosphere will rise to the surface and form brand new oceanic crust made of basalt and gabbro. The best example of this process at work can be found at the Mid-Atlantic Ridge, which is Earth’s longest mountain range. There are two sets of divergent boundaries at the Mid-Atlantic Ridge: the North American-Eurasian plate boundary and South American-African plate boundary. Such a long spreading center along the ocean floor is responsible for the majority of Earth’s volcanism, but because it is deep underwater, it poses little to no risk to society.

Continental Rifts
The lithosphere does not only spread apart along mid-ocean ridges! Although the continental lithosphere can be up to 100 km thick, the movement of tectonic plates can pull apart the crust. As a result, this crust will thin over time, and the lower layers of the crust and the mantle will rise. These rocks undergo decompression melting, and basaltic magma will erupt from surface volcanoes. These regions are called continental rift valleys. Most continental rift valleys form at divergent boundaries where ocean basins have not yet opened. These places are sites of continental break-up, and we can see this process occurring today at the East African Rift.
Convergent Boundaries
Convergent boundaries that form between two plates of oceanic lithosphere or at a boundary between oceanic and continental lithosphere will result in the subduction of the denser oceanic lithosphere toward the mantle. Within the subduction zone, magma will form in the overlying plate due to flux melting.
The magma that forms at the subduction zone is initially mafic; however, if it rises toward a plate composed mostly of continental crust, it will become intermediate or even felsic in composition. Stratovolcanoes typically form at convergent boundaries. The Pacific Ring of Fire is dominated by subduction zones with these explosive volcanoes.
A description of the Pacific Ring of Fire along western North America is below:
- Subduction at the Middle American Trench creates volcanoes in Central America.
- The San Andreas Fault is a transform boundary.
- Subduction of the Juan de Fuca plate beneath the North American plate creates the Cascade volcanoes like Mount St. Helens, Mount Rainer, Mount Hood, and more.
- Subduction of the Pacific plate beneath the North American plate in the north creates the long chain of the Aleutian Islands volcanoes near Alaska.
Hot Spots

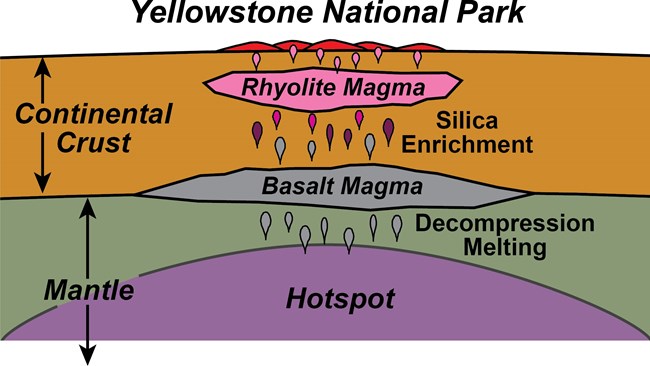
Hot spots are areas of partial melting deep within the mantle that rise and erupt at the surface. They are not associated with a plate boundary, and as such, the volcanic activity that results from hot spots is called intraplate volcanism (“intra” meaning within a plate). Hot spots may form brand new islands if they erupt new material over oceanic lithosphere. These islands are usually just “the tip of the iceberg” as they are often very large shield volcanoes that have been formed by low-viscosity, mafic lava. Additionally, these islands form in chains because the lithosphere will move over millions of years due to plate motion, but the hot spot will stay in place. The most famous example of hot spot volcanism may be the Hawaiian island chain, in which the hot spot remains active over Mauna Loa and Kilauea (above).
Some hot spots form beneath continental lithosphere. Although the partially-melted mantle composition is ultramafic at depth, as it rises to the surface and mixes with the materials in within the continental crust, it becomes mafic, then intermediate. In some areas, it might even become felsic. This mixing of molten materials is a recipe for potential disaster! As the magma becomes more silica-rich, it also becomes more viscous with volatile gases, which make for a very explosive eruption. In the United States, there is one active continental hot spot volcano we are currently monitoring: the Yellowstone Caldera (above), which is likely to have a super-volcanic eruption in the next 100,000 years.
Backyard Geology: Sunset Crater National Monument Park
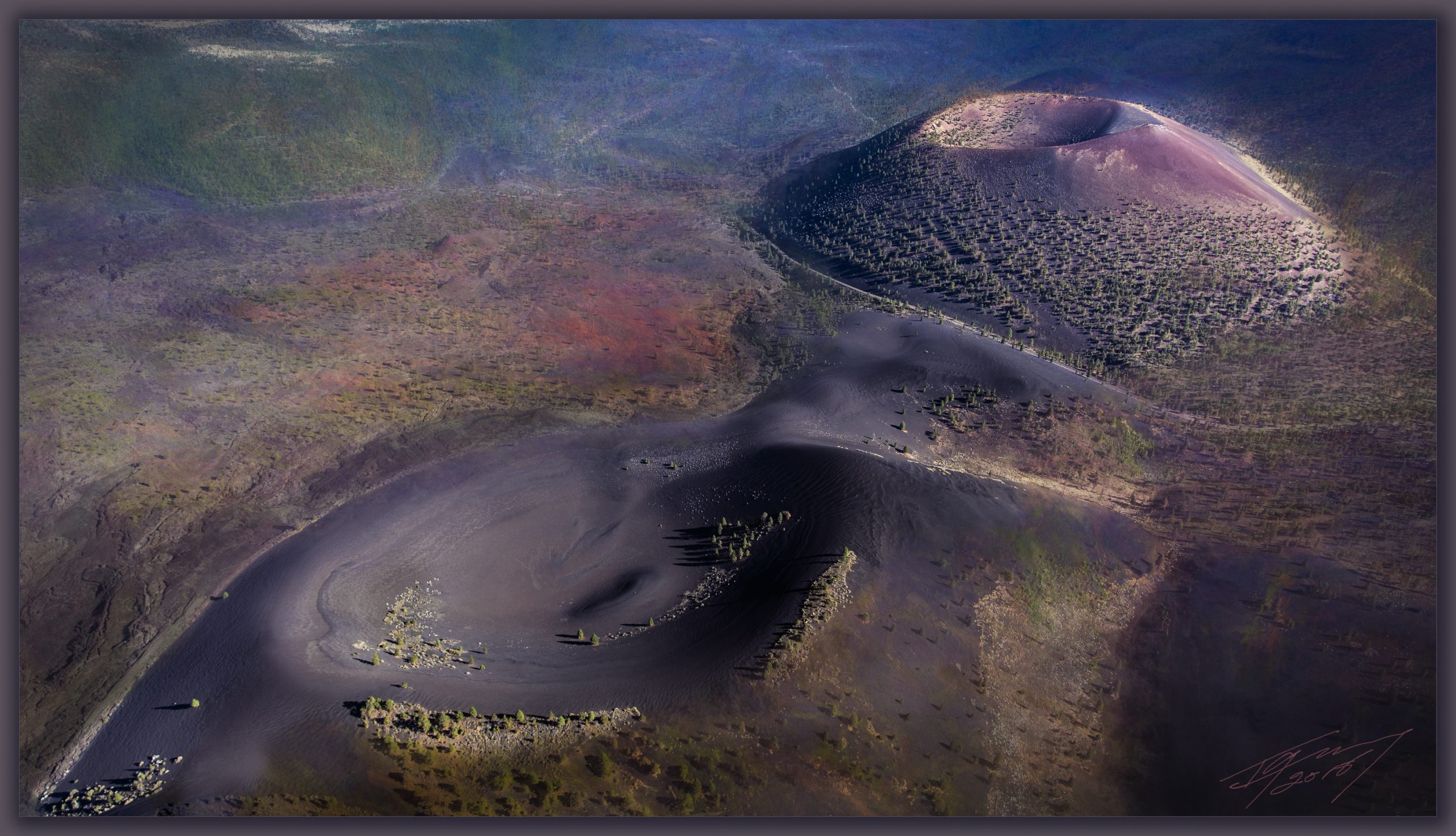
The Sunset Crater National Monument Park is a site of fairly recent (geologically-speaking!) volcanic activity. The park encompasses two cinder cone volcanoes; the Sunset Crater Volcano and Lenox Crater Volcano which have produced basaltic lavas and scoria from their previous eruptions. The last eruption from Sunset Crater was about 950 years ago, and it was said to result in lava fountains that were nearly 3 times higher than the Statue of Liberty. Even though Sunset Crater erupted in the past 1000 years, it is classified as extinct and it is not expected to erupt again as the hot spot has since moved eastward. We call it the “Sunset Crater” because mafic igneous rocks such as scoria have a lot of iron in them that easily rusts or oxidizes under Earth’s atmosphere from their original blackish-gray color to bright reds and purples, and pinks [10].
***See 5.8 for Text and Media Attributions
We may receive all the heat we need on the Earth's surface from sunlight, but if you dig a hole just a few feet deep, you might notice that the surrounding soil is much cooler. This happens because much of the sediment is insulated from the sun's energy. Nevertheless, if you had the ability to dig miles and miles into the crust, you would find that the temperature of the rock would start to significantly increase!

This increase in temperature with depth in the Earth's crust is called the geothermal gradient. In general, we can expect temperatures to increase about 25°C for each kilometer of depth. This sounds like a significant increase in heat, but once we pass the 100 km mark, temperatures really take off!
As we learned in the second chapter, the Earth's iron core is wickedly hot! Such heat powers plate tectonics, and when combined with other factors, it can lead to partial melting in the upper mantle. This melted, molten rock occasionally rises to the Earth's surface and when it does, watch out!
A volcano will form above a chamber or plume of magma. There is a wide variety of volcanoes, and some are more explosive than others. Molten lava is far from the only hazard; volcanic eruptions can cause massive damage with ashfall, pyroclastic flows, toxic gases, and deadly landslides. There are even a few volcanoes considered so dangerous that they are termed "supervolcanoes", and they have the potential to drastically impact society for generations.
Although volcanoes are hazardous, they are a continuous boon for the global economy. The precious minerals and useful rocks formed by volcanic processes have benefited civilization for thousands of years. In the modern economy, we use volcanism as a source of alternative energy and igneous rocks in trades ranging from construction to cosmetics.
Learning Objectives
- Describe the size, shape, and eruption style of volcanoes and how they form/
- Identify the major volcanic hazards and their effects on humans and property.
- Explain how scientists monitor volcanoes and where volcanoes can be found.
- Explain how volcanic processes benefit society.
As has already been described, igneous rocks are classified into four categories: felsic, intermediate, mafic, and ultramafic, based on either their chemistry or their mineral composition. The diagram in Figure 4.4.1 can be used to help classify igneous rocks by their mineral composition. An important feature to note on this diagram is the red line separating the non-ferromagnesian silicates in the lower left (K-feldspar, quartz, and plagioclase feldspar) from the ferromagnesian silicates in the upper right (biotite, amphibole, pyroxene, and olivine). In classifying intrusive igneous rocks, the first thing to consider is the percentage of ferromagnesian silicates. In most igneous rocks the ferromagnesian silicate minerals are clearly darker than the others, but it is still quite difficult to estimate the proportions of minerals in a rock.
Based on the position of the red line in Figure 4.4.1, it is evident that felsic rocks can have between 1% and 20% ferromagnesian silicates (the red line intersects the left side of the felsic zone 1% of the distance from the top of the diagram, and it intersects the right side of the felsic zone 20% of the distance from the top). Intermediate rocks have between 20% and 50% ferromagnesian silicates, and mafic rocks have 50% to 100% ferromagnesian silicates. To be more specific, felsic rocks typically have biotite and/or amphibole; intermediate rocks have amphibole and, in some cases, pyroxene; and mafic rocks have pyroxene and, in some cases, olivine.
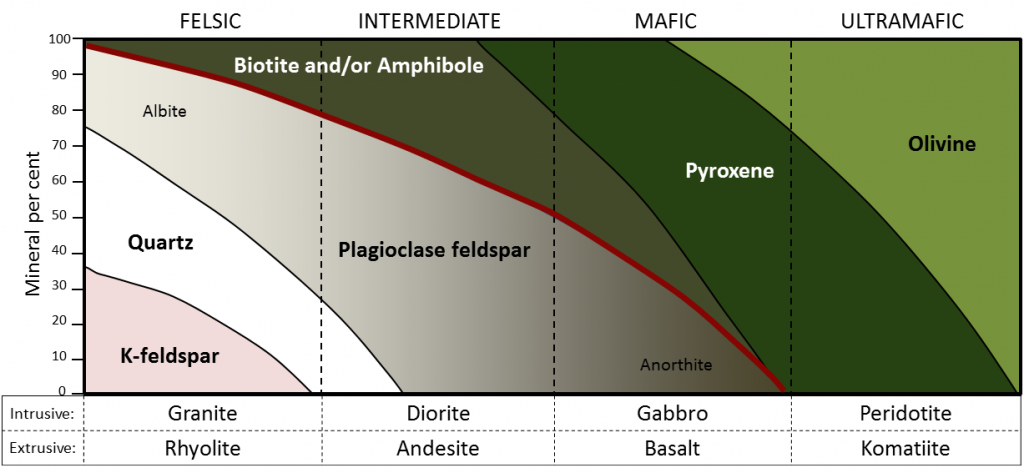
If we focus on the non-ferromagnesian silicates, it is evident that felsic rocks can have from 0% to 35% K-feldspar, from 25% to 35% quartz (the vertical thickness of the quartz field varies from 25% to 35%), and from 25% to 50% plagioclase (and that plagioclase will be sodium-rich, or albitic). Intermediate rocks can have up to 25% quartz and 50% to 75% plagioclase. Mafic rocks only have plagioclase (up to 50%), and that plagioclase will be calcium-rich, or anorthitic.
Exercise: Mineral proportions in igneous rocks

Figure 4.4.3 provides a diagrammatic representation of the proportions of dark minerals in light-colored rocks. You can use that when trying to estimate the ferromagnesian mineral content of actual rocks, and you can get some practice doing that by completing Exercise 3.6. Be warned! Geology students almost universally over-estimate the proportion of dark minerals.

Exercise: Proportions of ferromagnesian silicates
The four igneous rocks shown below have differing proportions of ferromagnesian silicates.
Igneous rocks are also classified according to their textures. The textures of volcanic rocks will be discussed in Chapter 4, so here we’ll only look at the different textures of intrusive igneous rocks. Almost all intrusive igneous rocks have crystals that are large enough to see with the naked eye, and we use the term phaneritic (from the Greek word phaneros meaning visible) to describe that. Typically that means they are larger than about 0.5 millimeters (mm) — the thickness of a strong line made with a ballpoint pen. (If the crystals are too small to distinguish, which is typical of most volcanic rocks, we use the term aphanitic (from the Greek word aphanos - unseen) The intrusive rocks shown in Figure 3.3.5 are all phaneritic, as are those shown in Exercise 3.6.
In general, the size of crystals is proportional to the rate of cooling. The longer it takes for a body of magma to cool, the larger the crystals can grow. It is not uncommon to see an intrusive igneous rock with crystals up to 1 centimeter (cm) long. In some situations, especially toward the end of the cooling stage, the magma can become water rich. The presence of liquid water (still liquid at high temperatures because it is under pressure) promotes the relatively easy movement of ions, and this allows crystals to grow large, sometimes to several centimeters (Figure 3.4.4). Finally, as already described, if an igneous rock goes through a two-stage cooling process, its texture will be porphyritic (Figure 4.3.7).

The world's greatest Mass Extinction event that occurred around 252 million years ago and resulted in the loss of 95% of ocean life and 70% of life on land.
material moves as a mass along a sloping surface
The study and monitoring of volcanoes.
Fine particles, liquid droplets, or gases that are ejected into the atmosphere by man-made pollution or volcanic eruptions. Aerosols have the ability to reflect sunlight back toward space and cause the planet to experience colder temperatures.
volcanic activity that occurs between active tectonic plate boundaries.
Geology is an umbrella science that encompasses all biological, chemical, and physical processes that act on the planet and make up our world. In the same way that the human body can be divided into systems (circulatory, digestive, endocrine, etc.), the Earth can be described as a complex entity that encompasses many different systems all acting on one another. There are 5 main systems, or spheres, found on Earth: geosphere, biosphere, hydrosphere, atmosphere, and cryosphere.
Geosphere ("geo" = Earth)
The geosphere encompasses all physical material that makes up the interior and surface of the Earth. This includes all rocks and minerals, but is also includes the active processes that link these rocks and minerals together. While many tend to focus on the three types of rocks: igneous, sedimentary, and metamorphic, the forces of plate tectonics and weathering actively change rocks from one type to another. This system is referred to as the Rock Cycle. This is the focus of most physical geology courses and will be the main framework for this text. Because the geosphere describes the physical structure of the Earth, it is integral to all other spheres as well.
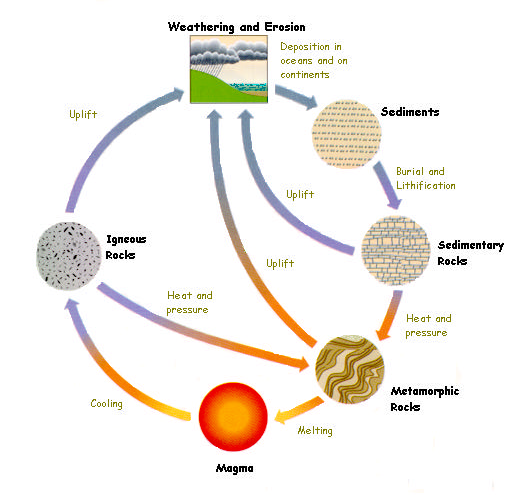
Biosphere ("bio" = life)
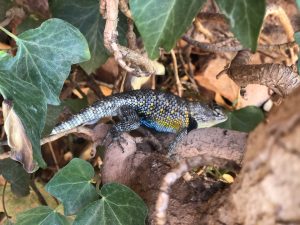
The biosphere is made up of all living things, as well as the ecosystems that support that life. Obviously, life can be found in many different places, from the tops of mountains to the bottoms of oceans, in wet and dry places, in cold and hot environments. The biosphere therefore overlaps and interacts with all other spheres.
Hydrosphere ("hydro" = water)
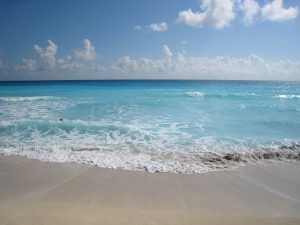
The Earth has been described as the “Blue Marble” in space due to the presence of enormous amounts of water on its surface. All of the liquid water found on earth makes up the hydrosphere. As water flows on the Earth’s surface, it changes landscapes and nourishes life, and interacts with all other spheres.
Atmosphere ("atmo" = vapor)
All of the gases that circulate around and surround the earth are a part of the atmosphere. This includes the air we breathe, the gases that are responsible for global weather and climate, as well as the ones that burn up meteorites as they plummet toward the Earth’s surface. The atmosphere is further broken into layers: troposphere, stratosphere, mesosphere, thermosphere, and exosphere.
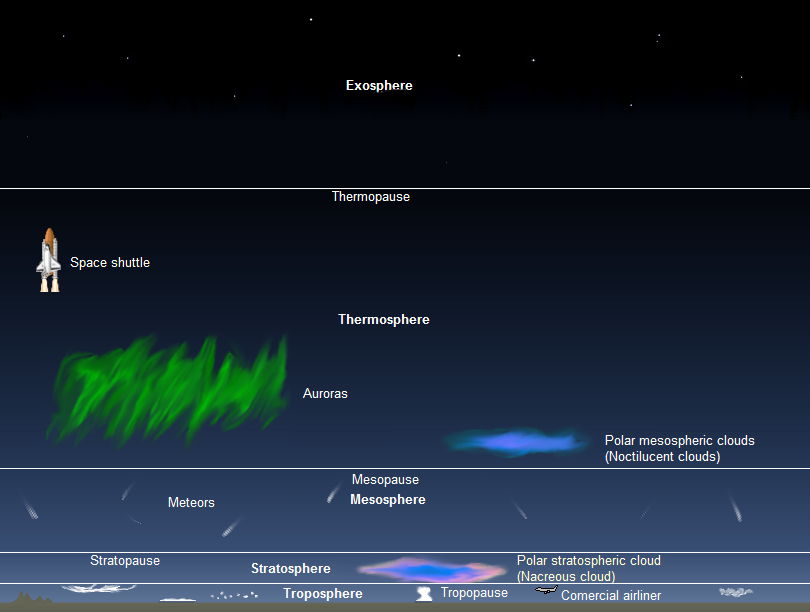
Cryosphere ("cryo" = frozen)
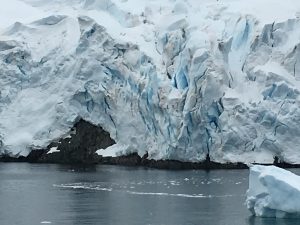
Cryo- comes from a Greek word meaning "frost." The cryosphere encompasses all of frozen water on the Earth. Typically found at high elevations and at the poles, ice can have dramatic impacts on landscapes (geosphere), provide sources of liquid water (hydrosphere), and support ecosystems for living things (biosphere).
Integrated systems
While it is easier to look at each system independently, they are all integrated on the Earth. Each one is constantly acting and interacting with another on our dynamic planet.
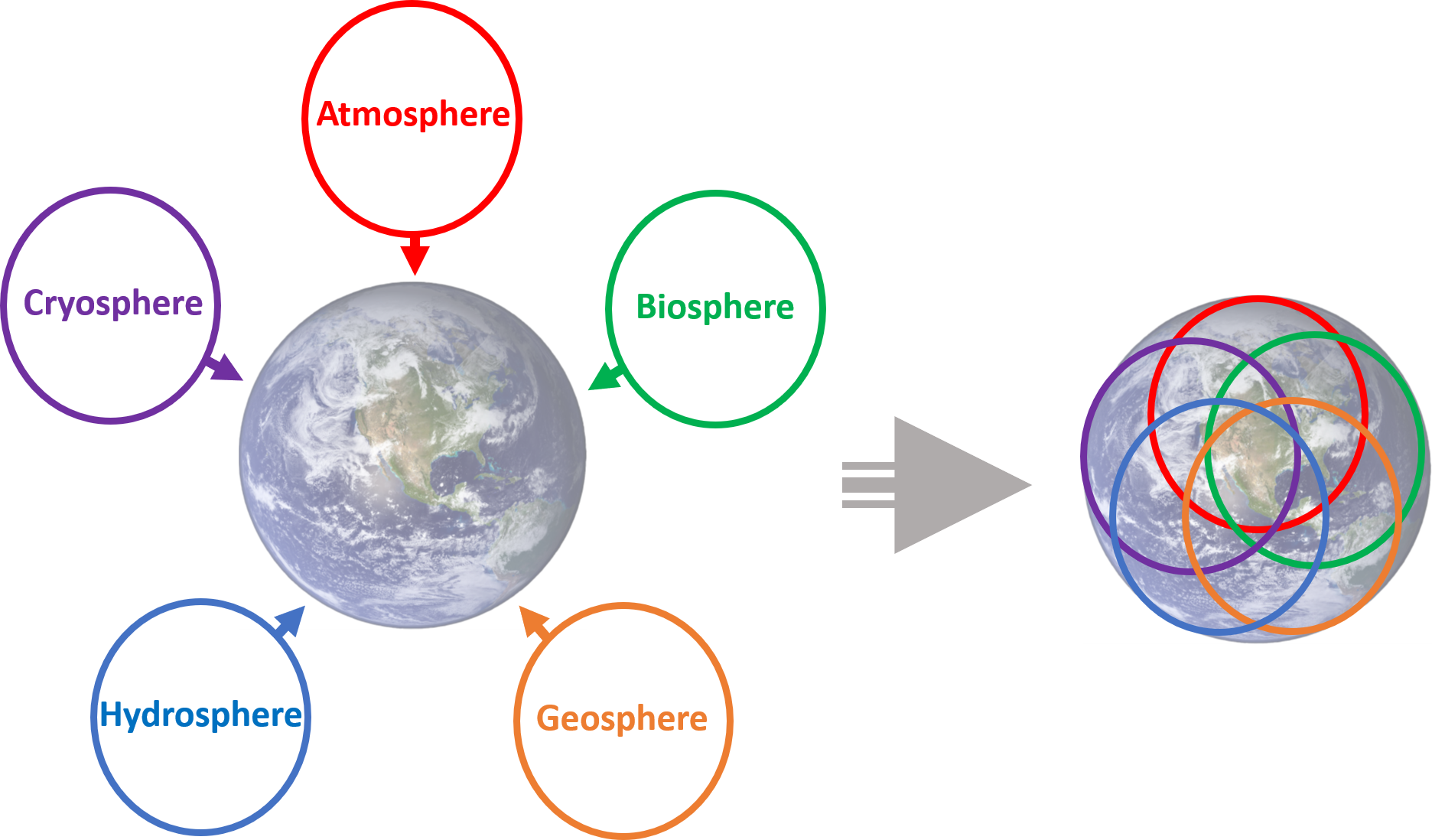
H2O, or the water molecule, is constantly changing. It is the only substance on Earth that is present in all states of matter - solid, liquid, and gas. The cycling of water throughout the Earth, also called the hydrologic cycle, describes how water moves through various environments on Earth. The largest reservoir of water is in the ocean, and it exists there as a liquid. Through the process of evaporation, it becomes a gas. At that point, it may crystallize as a solid (snow or ice) and deposit on land. It could then melt, forming a liquid again, and run off the landforms, eternally shaping the Earth. It can be taken up into plants, aiding in photosynthesis, and released as a gas through the process of transpiration. The possibilities of pathways for water to travel on Earth are endless.
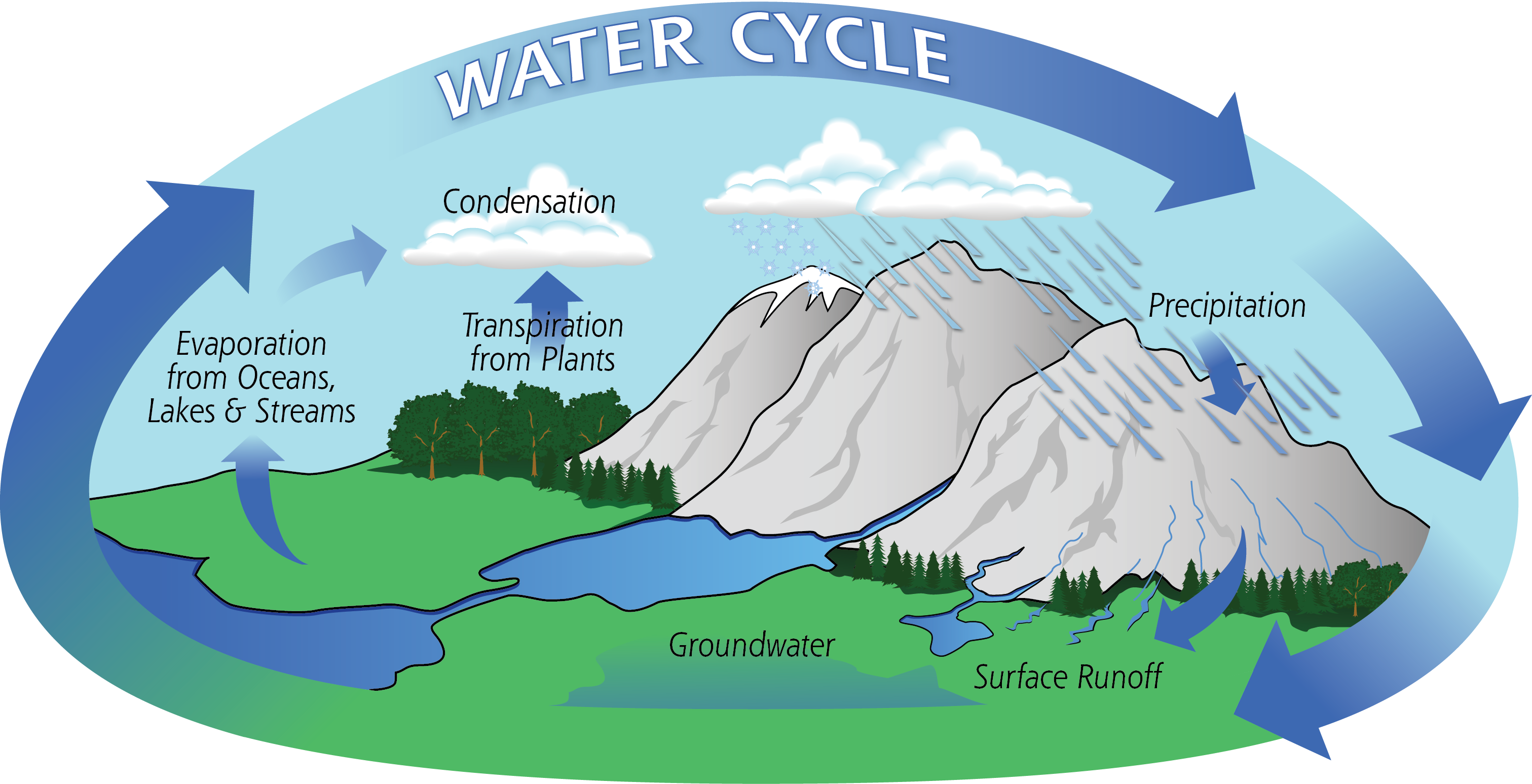
Backyard Geology: Spheres intersect for one of the 7 Natural Wonders of the World
Arizona is known as the "Grand Canyon State" because of the dramatic landscape that unfolds at the intersection of brightly colored flat-lying sedimentary rocks and a dynamic river system. This landscape evolved over millions of years while the underlying rocks were uplifted by Plate Tectonics, and the Colorado River cut down through soft sedimentary rocks..
***See 1.5 for Text and Media Attributions
Image by James St. John (Flickr), CC BY 2.0
molten rock that can be found beneath the Earth's surface.
scientists that study seismic waves
A measure of the amount of matter, or atoms, that a given object or material with finite boundaries contains. Your mass will not change from planet to planet.
Scientific Method in Geosciences: Multiple hypotheses, Multiple modes of Inquiry
How is science made in Earth Sciences? Why should you care? Even if you are not interested in becoming an Earth scientist, or any kind of scientist, understanding how science works is a source of empowerment. This is because science and scientific thinking are at the heart of our modern way of life and they influence every aspect of our lives. Understanding how science works can help you discern fact from fiction and inform your life choices and political decisions.
Modern science is based on the scientific method, the idea in science that phenomena and ideas need to be scrutinized using hypothesizing, experimentation, and analysis. This can eventually result in a consensus or scientific theory.
The scientific method, is a procedure that follows these steps:
- Formulate a question or observe a problem
- Apply objective experimentation and observation
- Analyze collected data and Interpret results.
- Devise an evidence-based theory.
- Submit findings to peer review.
Let's see how scientific inquiry works in Earth science.
1. Observation, problem, or research question
The procedure begins when scientists identify a problem or research question, such as a geological phenomenon that is not well explained in the scientific community’s collective knowledge. This step usually involves reviewing the scientific literature to establish what is known and to consult previous studies related to the question.
Earth scientists can study processes they cannot observe directly, because they happen over long periods of time, or happened long ago, or happen in a remote location. An example of the last one would be the Earth's core. To circumvent these challenges, Earth scientists have developed strategies to test their hypothesis, these strategies make up the scientific method of geosciences.
2. Hypothesis
Once the scientists define the problem or question, they propose a possible answer, a hypothesis: A proposed explanation for an observation that can be tested. Hypotheses must be testable and falsifiable.
To test hypotheses, scientists use methods drawn from other sciences such as chemistry, physics, biology, or even engineering.
3. Testing Hypotheses: Experiments and Revisions

The next step is developing an experiment: A test of an idea in which new information can be gathered to either accept or reject a hypothesis.
Earth scientists conduct classic experiments in the lab, however, an experiment can take other forms such as:
- Observing natural processes and their products in the field and comparing them to those found in the rock record. E.g., A sedimentologist studies how wind moves and forms dunes and different ripples in a desert. This knowledge helps her to interpret ripple structures found in rocks, and even to interpret dunes and aeolian processes from images collected on Mars!
- Studying changes across time or space. E.g., an atmospheric scientist analyzes how the composition of the atmosphere has changed since we started measuring it.
- Using physical models. This is more akin to the "classic experiment". E.g., a team of scientists build a model for landslides using a long and steep ramp and flushing down different materials, and changing the ramp angle.
- Using computer models. E.g., climatologists develop computational models to study the climate system and make predictions. These scientific models undergo rigorous scrutiny and testing by collaborating and competing groups of scientists around the world.
- Considering multiple lines of evidence. To establish a scientific finding, all lines of evidence must converge, that means that all the results you collect using different methods must agree with the finding, the math must be sound, and the methods must be thoroughly described.
Regardless of what form an experiment takes, it always includes the systematic gathering of objective data. The scientists interpret this data to determine whether it contradicts or supports the hypothesis. If the results contradict the hypothesis, then scientists can revise it and test it again. When a hypothesis holds up under experimentation, it is ready to be shared with other experts in the field. The findings are scrutinized by the scientific community through the process of peer review.
4. Peer review, publication, and replication
Science is a social process. Scientists share the results of their research in conferences and by publishing articles in scientific journals, such as Science and Nature. Reputable journals and academic outlets will not publish an experimental study until they have determined its methods are scientifically rigorous and the evidence supports the conclusions. Before they publish the article, scientific experts in the field scrutinize the methods, results, and discussion; the peer review process. Once an article is published, other scientists may attempt to replicate the results. This replication is necessary to confirm the reliability of the study’s reported results. A hypothesis that seemed compelling in one study might be proven false in studies conducted by other scientists. New technology can be applied to published studies, which can aid in confirming or rejecting once-accepted ideas and/or hypotheses.
Backyard Geology: Wildfire in Saguaro National Park
Arizona ranks high in the number of individual fires, as well as acres burned, every year. In 2020, Arizona ranked 3rd out of all states in both categories, with 2,524 individual fires and 978,568 acres burned. Below are the top 10 states for wildfires:
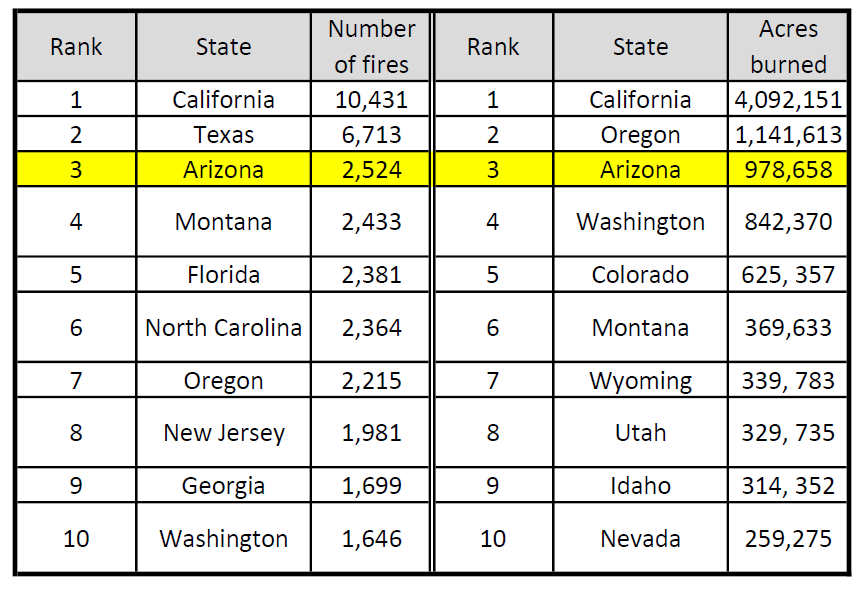
Why does Arizona have so many fires? Wildfire risk depends on many factors, including temperature, soil moisture, and the presence of vegetation (fuel) to burn. Certainly the dry, hot climate plays a role, and climate change intensifies drought conditions, but what contributes to the fuel available to burn?
In Saguaro National Park, an invasive noxious weed, called buffelgrass is changing the natural ecosystem and increasing the park's susceptibility to wildfire. Buffelgrass, unlike native vegetation, evolved with fire and thrives under repeated burning. It outcompetes native vegetation for resources, supplies material that can be readily burned, and connects areas subjected to wildfires. Steps have been taken to remove buffelgrass, including physical weeding and also using herbicides. Some areas are remote, and herbicides are applied by helicopter. What effect does this application have?
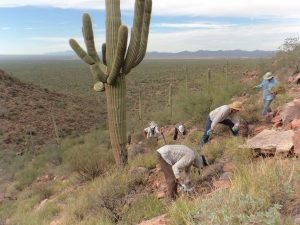
What geologists do? The United States Geological Survey (USGS) conducted a study to determine the effects of aerial dispersal of herbicide for buffelgrass control.
- Research Question: What are the potential transport and effects to aquatic ecosystems of the herbicides used for buffelgrass removal?
- Hypothesis: Herbicides will move into water environments and potentially be harmful to ecosystems and humans.
- Experiment: Three watersheds, treated with different amounts of herbicide, were regularly sampled to determine residual amounts of herbicides present in soil and water.
- Publication of results: While no water concentrations exceeded published criteria for human health or aquatic life, the authors acknowledge that it is a complex system that necessitates future study.
5. Theory development

In casual conversation, the word theory implies guesswork or speculation. In the language of science, an explanation or conclusion made into a carries much more weight because experiments support it and the scientific community widely accepts it. A hypothesis that has been repeatedly confirmed through documented and independent studies eventually becomes accepted as a scientific theory.
While a hypothesis provides a tentative explanation before an experiment, a theory is the best explanation after being confirmed by multiple independent experiments. Confirmation of a theory may take years, or even longer. For example, the scientific community initially dismissed the Continental Drift hypothesis first proposed by Alfred Wegener in 1912 (see Chapter 2). After decades of additional evidence collection by other scientists using more advanced technology, Wegener’s hypothesis was used as the framework and revised as the theory of Plate Tectonics.
In biology, theory of evolution by natural selection is another example. Originating from the work of Charles Darwin in the mid-19th century, the theory of evolution has withstood generations of scientific testing for falsifiability. It has been updated and revised to accommodate knowledge gained by using modern technologies, but the latest evidence continues to support the theory of evolution.
Science in Many Cultures
We have covered the scientific method of the geosciences. However, science is a human endeavor. For generations, Indigenous peoples have accrued empirical knowledge of the natural world, including the lithosphere. Greg Cajete uses the name Native Science to refer to "the collective heritage of human experience with the natural world" (Cajete, 2000). Geologic expertise served tribal peoples in many of the same ways that Western geology serves modern civilizations.
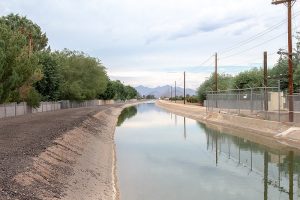
For example, Native Americans in Cascadia record volcanic events in stories, this transmits cross-generational awareness of volcanic hazards; the Muiscas in Colombia knew how to find, mine and process the gold to make beautiful art pieces; and the Puebloans of the North American Southwest managing limited water resources using an impressive net of canals that we still use for our benefit. Western and Native scientists can collaborate to further our understanding of the Earth systems and to remember how to live well on our planet.
Science is a dynamic process. Technological advances, breakthroughs in interpretation, and new observations continuously refine our understanding of Earth. We will never stop learning about our Earth. As new findings are published, we must revise and update our scientific knowledge and discard ideas that are proven false by new observations. Science is a living entity.
In conclusion, Earth scientists do not use a single, all-encompassing "scientific method". Instead, multiple modes of inquiry respond to the complexity and spatial and temporal scales of Earth systems.
"The unique thing about the geosciences is that the knowledge, skills, and methods are brought together, refined, and evolved over time to make them most suitable for understanding the complex processes of Earth, its working in the past and the present, and its likely behavior in the future." (Manduca and Kastens, 2012).
Key Takeaways
- Earth scientists work on challenging problems that face humanity on topics such as climate change, human impacts on Earth, and hazards to humans.
- Besides the classical laboratory experiment, Earth scientists construct models or use indirect methods to study the Earth.
- Scientific results are not valid or useful unless other scientists can reproduce them. Research results undergo scrutiny by the community before and after being published.
- The study of geological and environmental issues requires multiple disciplines and the interplay of multiple methods.
- Scientific thinking advances through collaboration and community.
GeoEthics

Geoscientists must act in ethical ways to contribute to the welfare of human beings. The saying "with great knowledge comes great responsibility" holds true for Earth and environmental scientists. Earth scientist must uphold high standards in research and conduct. The research and reflection upon the values which underpin behaviors and practices between humans and Earth systems is the arena of the "Geoethics" (Di Capua and Peppoloni, 2019). The International Association for Promoting Geoethics (IAPG) provide tools to "understand the complex relationship between human action on ecosystems and the decisions geoscientists make in the discipline that impact society, including improving the awareness of professionals, students, decision-makers, media operators, and the public on an accountable and ecologically sustainable development." Source: https://www.geoethics.org/geoethics-school
All of us, human beings, have responsibilities to Earth. We do not exist apart from this planet and our behaviors impact other people, other species, the larger biosphere, hydrosphere, atmosphere, and lithosphere. Our actions impact the Earth system. As you progress in your learning, strive to think critically and to identify ethical issues. Do not be afraid to question and discuss your observations with your instructor and with peers. Just remember to do so respectfully, considering other points of view and practicing active listening.
The world's longest mountain chain found beneath the Atlantic ocean between North America, South America and Europe and Africa. The Mid-Atlantic Ridge is an example of a mid-ocean ridge, or MOR.
A volcano that has erupted at least once over the past 10,000 years and has the possibility of erupting in the future.
earthquakes that precede larger earthquakes in the same location
The solidification of loose sediment materials as solid sedimentary rock through compacting pressures and cementation.
The process in which gravity and the pressure from overlying layers of rock cause loose sediments to press together.
The process in which solid rock melts upon experiencing a sudden decrease in pressure, but not temperature.
a process in which solid rock melts because volatiles such as water vapor have caused its melting point to decrease.
Originating from an iron and magnesium-rich magma/lava composition.
A magnesium and iron rich rock that contains very little silica.
Scientific Method in Geosciences: Multiple hypotheses, Multiple modes of Inquiry
How is science made in Earth Sciences? Why should you care? Even if you are not interested in becoming an Earth scientist, or any kind of scientist, understanding how science works is a source of empowerment. This is because science and scientific thinking are at the heart of our modern way of life and they influence every aspect of our lives. Understanding how science works can help you discern fact from fiction and inform your life choices and political decisions.
Modern science is based on the scientific method, the idea in science that phenomena and ideas need to be scrutinized using hypothesizing, experimentation, and analysis. This can eventually result in a consensus or scientific theory.
The scientific method, is a procedure that follows these steps:
- Formulate a question or observe a problem
- Apply objective experimentation and observation
- Analyze collected data and Interpret results.
- Devise an evidence-based theory.
- Submit findings to peer review.
Let's see how scientific inquiry works in Earth science.
1. Observation, problem, or research question
The procedure begins when scientists identify a problem or research question, such as a geological phenomenon that is not well explained in the scientific community’s collective knowledge. This step usually involves reviewing the scientific literature to establish what is known and to consult previous studies related to the question.
Earth scientists can study processes they cannot observe directly, because they happen over long periods of time, or happened long ago, or happen in a remote location. An example of the last one would be the Earth's core. To circumvent these challenges, Earth scientists have developed strategies to test their hypothesis, these strategies make up the scientific method of geosciences.
2. Hypothesis
Once the scientists define the problem or question, they propose a possible answer, a hypothesis
hypotheses to gather data from multiple lines. To test hypotheses, scientists use methods drawn from other sciences such as chemistry, physics, biology, or even engineering.
3. Testing Hypotheses: Experiments and Revisions

The next step is developing an <span aria-describedby="tt" data-cmtooltip="
Earth scientists conduct classic experiments in the lab, however, an
An experiment can take other forms such as:
- Observing natural processes and their products in the field and comparing them to those found in the rock record. E.g., A sedimentologist studies how wind moves and forms dunes and different ripples in a desert. This knowledge helps her to interpret ripple structures found in rocks, and even to interpret dunes and aeolian processes from images collected on Mars!
- Studying changes across time or space. E.g., an atmospheric scientist analyzes how the composition of the atmosphere has changed since we started measuring it.
- Using physical models. This is more akin to the "classic experiment". E.g., a team of scientists build a model for landslides using a long and steep ramp and flushing down different materials, and changing the ramp angle.
- Using computer models. E.g., climatologists develop computational models to study the climate system and make predictions. These scientific models undergo rigorous scrutiny and testing by collaborating and competing groups of scientists around the world.
- Considering multiple lines of evidence. To establish a scientific finding, all lines of evidence must converge, that means that all the results you collect using different methods must agree with the finding, the math must be sound, and the methods must be thoroughly described.
Regardless of what form an <span aria-describedby="tt" data-cmtooltip="
">experiment takes, it always includes the systematic gathering of <span aria-describedby="tt" data-cmtooltip="
">objective data. The scientists interpret this data to determine whether it contradicts or supports the <span aria-describedby="tt" data-cmtooltip="
">hypothesis (step 2). If the results contradict the hypothesis, then scientists can revise it and test it again. When a <span aria-describedby="tt" data-cmtooltip="
">hypothesis holds up under experimentation, it is ready to be shared with other experts in the field. The findings are scrutinized by the scientific community through the process of peer review.
Backyard Geology: Arizona Wildfire Study in Saguaro National Park
Arizona ranks high in the number of individual fires, as well as acres burned, every year. Below are the top 10 states for wildfires:
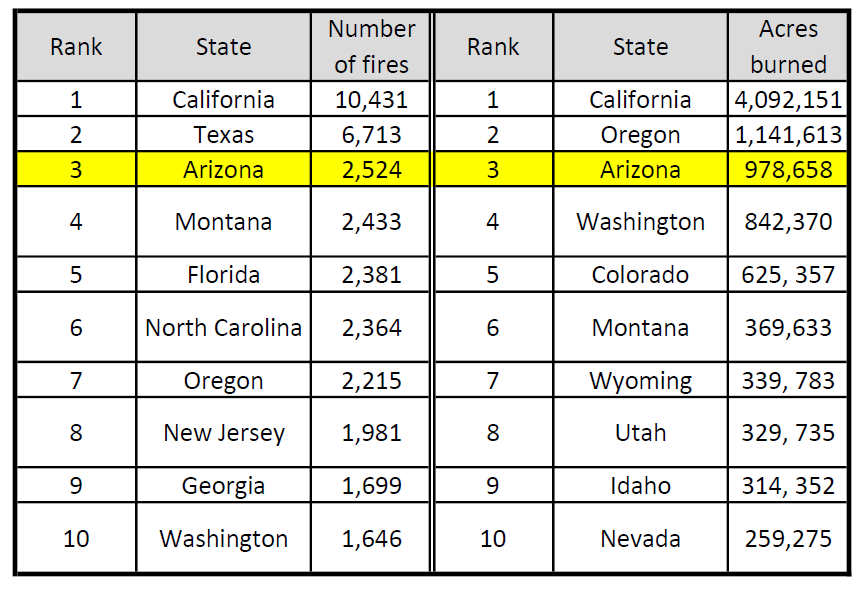
In 2020, Arizona ranked 3rd out of all states in both categories, with 2,524 individual fires and 978,568 acres burned. Why does Arizona have so many fires? Wildfire risk depends on many factors, including temperature, soil moisture, and the presence of vegetation (fuel) to burn. Certainly the dry, hot climate plays a role, and climate change intensifies drought conditions, but what contributes to the fuel available to burn?
It was determined in a previous study that invasive buffelgrass is fire-adapted, meaning it establishes, persists, and spreads following fire. It also outcompetes native vegetation for resources, supplies material that can be readily burned, and connects areas subjected to wildfires. Therefore, can we control or remove buffelgrass in the Arizona wilderness?
- Research Question: Invasive buffelgrass contributes to wildfires, can it be controlled or removed in wilderness areas?
- Hypothesis: If buffelgrass can be controlled, the intensity and interconnectedness of wildfires will lessen and cause less devastation.
- Experiment: Herbicides were used to control dense patches of buffelgrass in remote areas. The herbicide was applied with the use of helicopters.
- Results:
4. Peer review, publication, and replication
Science is a social process. Scientists share the results of their research in conferences and by publishing articles in scientific journals, such as Science and Nature. Reputable journals and academic outlets will not publish an experimental study until they have determined its methods are scientifically rigorous and the evidence supports the conclusions. Before they publish the article, scientific experts in the field scrutinize the methods, results, and discussion; the peer review process. Once an article is published, other scientists may attempt to replicate the results. This replication is necessary to confirm the reliability of the study’s reported results. A <span aria-describedby="tt" data-cmtooltip="
">hypothesis that seemed compelling in one study might be proven false in studies conducted by other scientists. New technology can be applied to published studies, which can aid in confirming or rejecting once-accepted ideas and/or <span aria-describedby="tt" data-cmtooltip="
">hypotheses.
5. Theory development

In casual conversation, the word <span aria-describedby="tt" data-cmtooltip="
">theory implies guesswork or speculation. In the language of science, an explanation or conclusion made into a <span aria-describedby="tt" data-cmtooltip="
">theory carries much more weight because experiments support it and the scientific community widely accepts it. A <span aria-describedby="tt" data-cmtooltip="
">hypothesis that has been repeatedly confirmed through documented and independent studies eventually becomes accepted as a scientific <span aria-describedby="tt" data-cmtooltip="
">theory.
While a <span aria-describedby="tt" data-cmtooltip="
">hypothesis provides a tentative explanation before an <span aria-describedby="tt" data-cmtooltip="
">experiment, a <span aria-describedby="tt" data-cmtooltip="
">theory is the best explanation after being confirmed by multiple independent experiments. Confirmation of a <span aria-describedby="tt" data-cmtooltip="
">theory may take years, or even longer. For example, the scientific community initially dismissed the <span aria-describedby="tt" data-cmtooltip="
">continental drift <span aria-describedby="tt" data-cmtooltip="
">hypothesis first proposed by Alfred Wegener in 1912 (see Chapter 2). After decades of additional evidence collection by other scientists using more advanced technology, Wegener’s <span aria-describedby="tt" data-cmtooltip="
">hypothesis was accepted and revised as the <span aria-describedby="tt" data-cmtooltip="
">theory of <span aria-describedby="tt" data-cmtooltip="
The <span aria-describedby="tt" data-cmtooltip="
">theory of evolution by natural selection is another example. Originating from the work of Charles Darwin in the mid-19th century, the <span aria-describedby="tt" data-cmtooltip="
">theory of evolution has withstood generations of scientific testing for falsifiability. It has been updated and revised to accommodate knowledge gained by using modern technologies, but the latest evidence continues to support the <span aria-describedby="tt" data-cmtooltip="
">theory of evolution.
Science
We have covered the scientific method of the geosciences. However, science is a human endeavor. For generations, Indigenous peoples have accrued empirical knowledge of the natural world, including the lithosphere. Greg Cajete uses the name Native Science to refer to "the collective heritage of human experience with the natural world" (Cajete, 2000). Geologic expertise served tribal peoples in many of the same ways that Western geology serves modern civilizations. For example, Native Americans in Cascadia record volcanic events in stories, this transmits cross-generational awareness of volcanic hazards; the Muiscas in Colombia knew how to find, mine and process the gold to make beautiful art pieces; and the Puebloans of the North American Southwest managing limited water resources using an impressive net of canals that we still use for our benefit. Western and Native scientists can collaborate to further our understanding of the Earth systems and to remember how to live well on our planet.
Science is a dynamic process. Technological advances, breakthroughs in interpretation, and new observations continuously refine our understanding of Earth. We will never stop learning about our Earth. As new findings are published, we must revise and update our scientific knowledge and discard ideas that are proven false by new observations. Science is a living entity.
In conclusion, Earth scientists do not use a single, all-encompassing "scientific method". Instead, multiple modes of inquiry respond to the complexity and spatial and temporal scales of Earth systems.
"The unique thing about the geosciences is that the knowledge, skills, and methods are brought together, refined, and evolved over time to make them most suitable for understanding the complex processes of Earth, its working in the past and the present, and its likely behavior in the future." (Manduca and Kastens, 2012).
Key Takeaways
- Earth scientists work on challenging problems that face humanity on topics such as climate change, human impacts on Earth, and hazards to humans.
- Besides the classical laboratory experiment, Earth scientists construct models or use indirect methods to study the Earth.
- Scientific results are not valid or useful unless other scientists can reproduce them. Research results undergo scrutiny by the community before and after being published.
- The study of geological and environmental issues requires multiple disciplines and the interplay of multiple methods.
- Scientific thinking advances through collaboration and community.
GeoEthics

Geoscientists must act in ethical ways to contribute to the welfare of human beings. The saying "with great knowledge comes great responsibility" holds true for Earth and environmental scientists. Earth scientist must uphold high standards in research and conduct. The research and reflection upon the values which underpin behaviors and practices between humans and Earth systems is the arena of the "Geoethics" (Di Capua and Peppoloni, 2019). The International Association for Promoting Geoethics (IAPG) provide tools to "understand the complex relationship between human action on ecosystems and the decisions geoscientists make in the discipline that impact society, including improving the awareness of professionals, students, decision-makers, media operators, and the public on an accountable and ecologically sustainable development." Source: https://www.geoethics.org/geoethics-school
All of us, human beings, have responsibilities to Earth. We do not exist apart from this planet and our behaviors impact other people, other species, the larger biosphere, hydrosphere, atmosphere, and lithosphere. Our actions impact the Earth system. As you progress in your learning, strive to think critically and to identify ethical issues. Do not be afraid to question and discuss your observations with your instructor and with peers. Just remember to do so respectfully, considering other points of view and practicing active listening.
originating from a feldspar and silica-rich magma/lava composition.
An alternative and renewable fuel source in which electricity is generated from the Earth's heat from volcanic activity below the surface.
Magma vs. Lava
You may have heard both magma and lava used to refer to molten rock. This can be confusing, especially since BOTH of these terms do apply to hot, melted rock. So what's the difference, anyway? It comes down to location. Magma is hot, molten rock that exists beneath the Earth's surface. It can be found in the crust right below a volcano or within the mantle. For a volcano to erupt, it must have a source of magma.
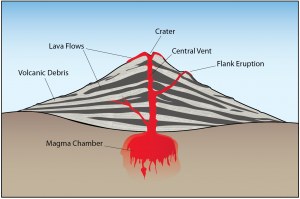
Lava, on the other hand, is only observed at the surface following a volcanic eruption. It is no different in composition from magma because it is sourced directly from a magma chamber. Besides erupting only at the surface, lava cools quickly because it is exposed to the Earth's atmosphere (or sometimes ocean!), and we see different igneous rock textures as a consequence. Igneous rocks from magma are coarse-grained or intrusive because they cool slowly, but those from lava are fine-grained, or extrusive.
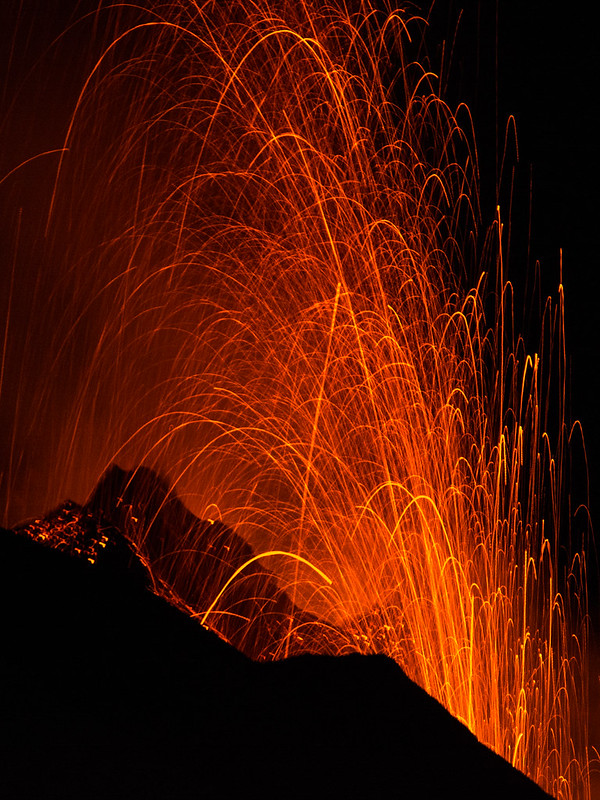
Melting Solid Rock
Magma and lava contain three components – melt, solids, and volatiles (dissolved gases). The liquid part, called melt, is made of ions from minerals that have already melted.
All you need to melt a solid rock is heat, right? Wrong! Most lava at volcanoes is around 700 to 1300 °C, which is typical of our upper mantle. However, our mantle as a whole is solid, so something else is required to cause rock to melt. That "something else" can be a sudden decrease in pressure or the introduction of liquid water, which will lower the melting point of rocks in the mantle.
Decompression Melting
Our mantle is solid, but under high temperatures and pressures, it flows over very long timescales in a process known as convection. Convection forms circular cells of movement for the rock within the mantle, and sometimes leads to the upwelling of hot mantle material at divergent plate boundaries and hot spots.
Rock is a bad conductor of heat, so as rock in the mantle rises with upwelling or convection, its temperature does not significantly change. Nevertheless, when that rock rises, the pressure of the rock does decrease because the depth decreases. When the hot mantle rock does not have sufficiently high pressures to keep itself solid, it starts to melt. This process of the rock melting due to a sudden change in pressure is called decompression melting, and it typically occurs at hot spots and divergent boundaries, such as the Mid-Atlantic Ridge [1].
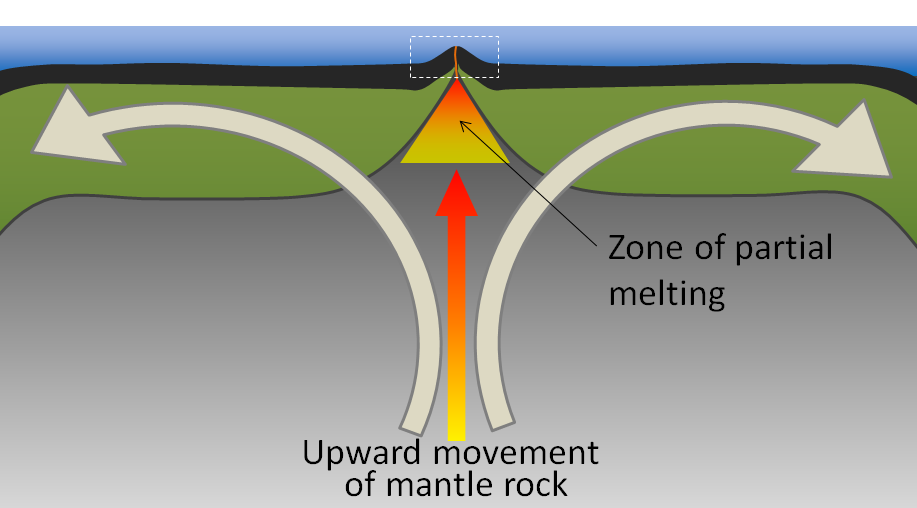
Flux Melting
At subduction zones along the Earth's lithosphere, the descending slab is always made of oceanic lithosphere. This slab contains some hydrated minerals that when exposed to elevated temperatures and pressures during subduction, will become released as volatile gases such as water vapor.
These volatile gases rise and interact with the overlying plate in the subduction zone. The addition of the volatiles does not change the pressure or temperature of the rock, but it does lower a property called the melting point. The decrease in melting point with those added volatiles suddenly makes it possible for the rocks in the subduction zone to melt at the pressures and temperatures they have been experiencing, which is why we observe volcanoes at this type of plate boundary. Magmas producing the volcanoes of the Ring of Fire, associated with the subduction zones bordering the Pacific Ocean, are a result of flux melting [1].
Magma Composition
https://www.youtube.com/watch?v=WojOleI9C2s
In 1980, Mount St. Helens blew up in the costliest and deadliest volcanic eruption in United States history. The eruption killed 57 people, destroyed 250 homes, and swept away 47 bridges. Mount St. Helens today still has minor earthquakes and eruptions, and now has a horseshoe-shaped crater with a lava dome inside. The dome is made of pb_glossary id="1812"]viscous[/pb_glossary] lava that oozes into place [1].
Volcanoes do not always erupt in the same way. Each volcanic eruption is unique, differing in size, style, and composition of the erupted material. One key to what makes the eruption unique is the chemical composition of the magma that feeds a volcano, which determines (1) the eruption style, (2) the type of volcanic cone that forms, and (3) the composition of rocks that are found at the volcano.
The words that describe the composition of igneous rocks also describe magma composition. Mafic magmas are low in silica and have darker magnesium (Mg), and iron (Fe)-rich minerals, such as olivine and pyroxene.
Felsic magmas are higher in silica and have lighter-colored minerals such as quartz and orthoclase feldspar.
The higher the amount of silica in the magma, the higher is its viscosity. Viscosity is a liquid’s resistance to flow or movement within the Earth or on its surface. Viscosity determines what the magma will do. Mafic magma is not very viscous and will flow smoothly to the surface.
https://www.youtube.com/watch?v=KgwfH3wkfA4
Volcanoes with a mafic composition will typically not have very explosive eruptions, but the lava erupted will be fast moving. This mafic lava often moves down mountainsides and cools rapidly into unique textures that are either ropey called "Pahoehoe" or rough and rocky called "A'a".
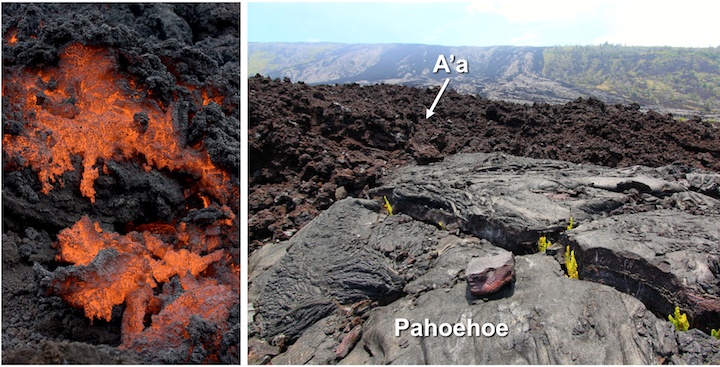
Felsic magma is very viscous, and it does not flow smoothly. Most felsic magma will stay deeper in the crust and will cool to form intrusive igneous rocks such as granite and diorite. If felsic magma rises into a magma chamber, it may be too viscous to move, so it tends to get stuck.
However, intermediate magma is also highly viscous, and it contains dissolved volatile gases. These gases become trapped by the magma, and the magma chamber begins to build in pressure. When the magma finally can erupt as lava, it does so very violently and explosively, as we have seen at Mount St. Helens.
As previously discussed in section 3.9, a rock is a collection of consolidated minerals. Rocks are grouped into three main categories based on how they form:
- Igneous: formed from the cooling and crystallization of molten rock.
- Sedimentary: formed when weathered fragments of other rocks are buried, compressed, and cemented together, or when minerals precipitate directly from solution
- Metamorphic: formed by alteration (due to heat, pressure, and/or chemical action) of a pre-existing igneous or sedimentary rock
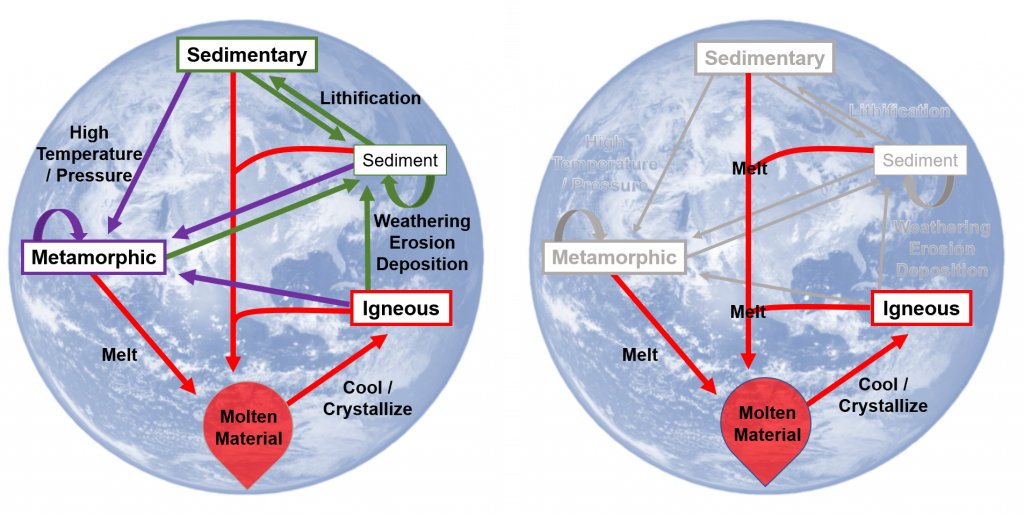
The focus of this chapter is on Igneous rocks, though it is important to remember this is just a piece of the rock cycle.
Learning Objectives
After carefully reading this chapter, completing the exercises within it, and answering the questions at the end, you should be able to:
- Describe the rock cycle and the types of processes that lead to the formation of igneous, sedimentary, and metamorphic rocks, and explain why there is an active rock cycle on Earth.
- Explain the concept of partial melting and describe the geological processes that lead to melting.
- Describe, in general terms, the range of chemical compositions of magmas.
- Discuss the processes that take place during the cooling and crystallization of magma, and the typical order of crystallization according to the Bowen reaction series.
- Explain how magma composition can be changed by fractional crystallization and partial melting of the surrounding rocks.
- Apply the criteria for igneous rock classification based on mineral proportions.
- Describe the origins of phaneritic, porphyritic, and pegmatitic rock textures.
- Identify plutons on the basis of their morphology and their relationships to the surrounding rocks.
- Explain the origin of a chilled margin.
a liquid's resistance to flow or movement
The process in which fluids, such as water, move into the pore spaces between loose sediments and - through chemical reactions - act as a bonding agent between the grains.
Time is the dimension that sets geology apart from most other sciences. Geological time is vast, and Earth has changed enough over that time that some of the rock types that formed in the past could not form today. Furthermore, as we’ve discussed, even though most geological processes are very, very slow, the vast amount of time that has passed has allowed for the formation of extraordinary geological features, as shown in Figure 8.0.1.

We have numerous ways of measuring geological time. We can tell the relative ages of rocks (for example, whether one rock is older than another) based on their spatial relationships; we can use fossils to date sedimentary rocks because we have a detailed record of the evolution of life on Earth; and we can use a range of isotopic techniques to determine the actual ages (in millions of years) of igneous and metamorphic rocks.
But just because we can measure geological time doesn’t mean that we understand it. One of the biggest hurdles faced by geology students—and geologists as well—in mastering geology, is to really come to grips with the slow rates at which geological processes happen and the vast amount of time involved.\
Learning Objectives
After carefully reading this chapter, completing the exercises within it, and answering the questions at the end, you should be able to:
- Apply basic geological principles to the determination of the relative ages of rocks.
- Explain the difference between relative and absolute age-dating techniques.
- Summarize the history of the geological time scale and the relationships between eons, eras, periods, and epochs.
- Understand the importance and significance of unconformities.
- Estimate the age of a rock based on the fossils that it contains.
- Describe some applications and limitations of isotopic techniques for geological dating.
- Use isotopic data to estimate the age of a rock.
- Describe the techniques for dating geological materials using tree rings and magnetic data.
- Explain why an understanding of geological time is critical to both geologists and the public in general.
Media Attributions
- © Steven Earle. CC BY.
Gases dissolved within magma or lava.
Mechanisms
Sea-level change has been a feature on Earth for billions of years, and it has important implications for coastal processes and both erosional and depositional features. There are three primary mechanisms of sea-level change, as described below.

Eustatic
Eustatic sea-level changes are global sea-level changes related to changes in the volume of glacial ice on land or changes in the shape of the seafloor caused by plate tectonic processes. For example, changes in the rate of mid-ocean spreading will change the seafloor's shape near the ridges, which affects sea level. Over the past 20,000 years, there have been approximately 125 meters (410 feet) of eustatic sea-level rise due to glacial melting. Most of that took place between 15,000 and 7,500 years ago during the significant melting phase of the North American and Eurasian Ice Sheets. At around 7,500 years ago, the rate of glacial melting and sea-level rise decreased dramatically, and since that time, the average rate has been in the order of 0.7 mm/year. Anthropogenic climate change led to an accelerating sea-level rise starting around 1870. Since that time, the average rate has been 1.1 mm/year, but it has been gradually increasing. Since 1992, the average rate has been 3.2 mm/year. (4)
Isostatic
Isostatic sea-level changes are local changes caused by subsidence or uplift of the crust related either to changes in the amount of ice on the land or to growth or erosion of mountains. Almost all of Canada and parts of the northern United States were covered in thick ice sheets at the peak of the last glaciation. Following the melting of this ice, there has been an isostatic rebound of continental crust in many areas. This ranges from several hundred meters of rebound in the central part of the Laurentide Ice Sheet (around Hudson Bay) to 100 m to 200 m in the peripheral parts of the Laurentide and Cordilleran Ice Sheets - in places such as Vancouver Island and the mainland coast of BC. Although the global sea level was about 130 m lower during the last glaciation, the glaciated regions were depressed at least that much in most places, and more than that in places where the ice was thickest. (7)
Tectonic
Tectonic sea-level changes are local changes caused by tectonic processes. The subduction of the Juan de Fuca Plate beneath British Columbia creates tectonic uplift (about 1 mm/year) along the western edge of Vancouver Island, although much of this uplift is likely to be reversed when the next sizeable subduction-zone earthquake strikes. (4)
Emergent and Submergent Coasts
Coastlines that have a relative fall in sea level, either caused by tectonics or sea-level change, are called emergent. Where the shoreline is rocky, with a sea cliff, waves refracting around headlands attack the rocks behind the point of the headland.
They may cut out the rock at the base forming a sea arch that may collapse to isolate the point as a stack. Rocks behind the stack may be eroded, and sand eroded from the point collects behind it, forming a tombolo, a sand strip that connects the stack to the shoreline. Where sand supply is low, wave energy may erode a wave-cut platform across the surf zone, exposed as bare rock with tidal pools at low tide. Sea cliffs tend to be persistent features as the waves cut away at their base, and higher rocks calve off by mass wasting. If the coast is emergent, these erosional features may be elevated compared to the wave zone. Wave-cut platforms become marine terraces, with remnant sea cliffs inland from them. (4)
Tectonic subsidence or sea-level rise produces a submergent coast. Features associated with submergence coasts include estuaries, bays, and river mouths flooded by the higher water. Fjords are ancient glacial valleys now flooded by post-Ice Age sea level rise. Barrier islands form parallel to the shoreline from the old beach sands, often isolated from the mainland by lagoons behind them. Some scientists hypothesize that barrier islands formed by rising sea levels as the ice sheets melted after the last ice age. Accumulation of spits and far offshore bar formations are also mentioned as formation hypotheses for barrier islands.
Estuaries and fiords commonly characterize coastlines in areas where there has been a net sea-level rise in the geologically recent past. This valley was filled with ice during the last glaciation, and there has been a net rise in sea level here since that time. Uplifted wave-cut platforms or stream valleys characterize coastlines in areas where there has been a net sea-level drop in the geologically recent past. Uplifted beach lines are another product of relative sea-level drop, although these are difficult to recognize in areas with vigorous vegetation.
Backyard Geology: Sea-level changes recorded in rocks
While it seems fairly obvious that there are no real coastlines in Arizona currently, their existence is preserved in rocks throughout the state. These ancient deposits imply that they were formed in a similar environment as the rocks of present day, so there must have been oceans and shorelines throughout Arizona in the past.
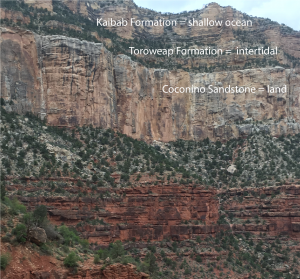
Figure 8.5.2 shows the three uppermost layers of the Grand Canyon. Though these layers were deposited millions of years ago, they preserve the environments that were present at their time of deposition. The Kaibab Formation, the youngest set of rocks found in the Grand Canyon, are a 270 million-year-old limestone that was deposited in a shallow marine (ocean) environment. The Toroweap Formation was formed in an intertidal zone, as sea-level changed several times. The older Coconino Sandstone is a 275 million-year-old wind-blown sand which forms a dramatic cliff in the present day. Together, these rock show that sea-level changed dramatically in just 5 million years, and much of Arizona was entirely under water!
Image by Karla Panchuk (CC BY 2.0)