5.4 Monitoring Volcanoes
Predicting Volcanic Eruptions
[Video Description: Images of molten lava at active volcanic craters. “Volcanoes are SO HOT right now. SO HOT that globally around 40 volcanoes erupt each month. Clip of hydrothermal vent. “Along with hundreds more erupting on the seafloor.” Video of lava. “EACH MONTH. And every eruption is an opportunity to learn about what’s happening deep, deep within the Earth.” Video of white volcanic gases. “In addition to lava and ash, these portals to the deep emit gases. Water, carbon and sulfur from within Earth provide valuable clues about how our planet works. Collecting these gases might look cool, but it’s the HOTTEST job in science.” Video of Jeep driving down the road. “By 2019, the Deep Carbon Observatory will TRIPLE the number of continuous volcanic gas monitoring stations worldwide, collecting real-time data in some of the most remote places on Earth.” World map of earthquakes, eruptions, and gas emissions. “When pieced together, this information helps reveal the story of deep carbon. Measuring these gases may even help us forecast future volcanic eruptions, and that’s not just a bunch of HOT AIR.” Video of erupting stratovolcano by a town.]
Scorching temperatures, toxic gases, and let’s not forget the volcanic bombs! Monitoring volcanoes is an extremely dangerous job, and it comes as no surprise that volcanology routinely appears among the top ten deadliest professions in the world! Nevertheless, there are scientists, as you have seen in the video above, who take the risk to monitor volcanoes because it may reduce the chance of a potential eruption taking a populated region by surprise.
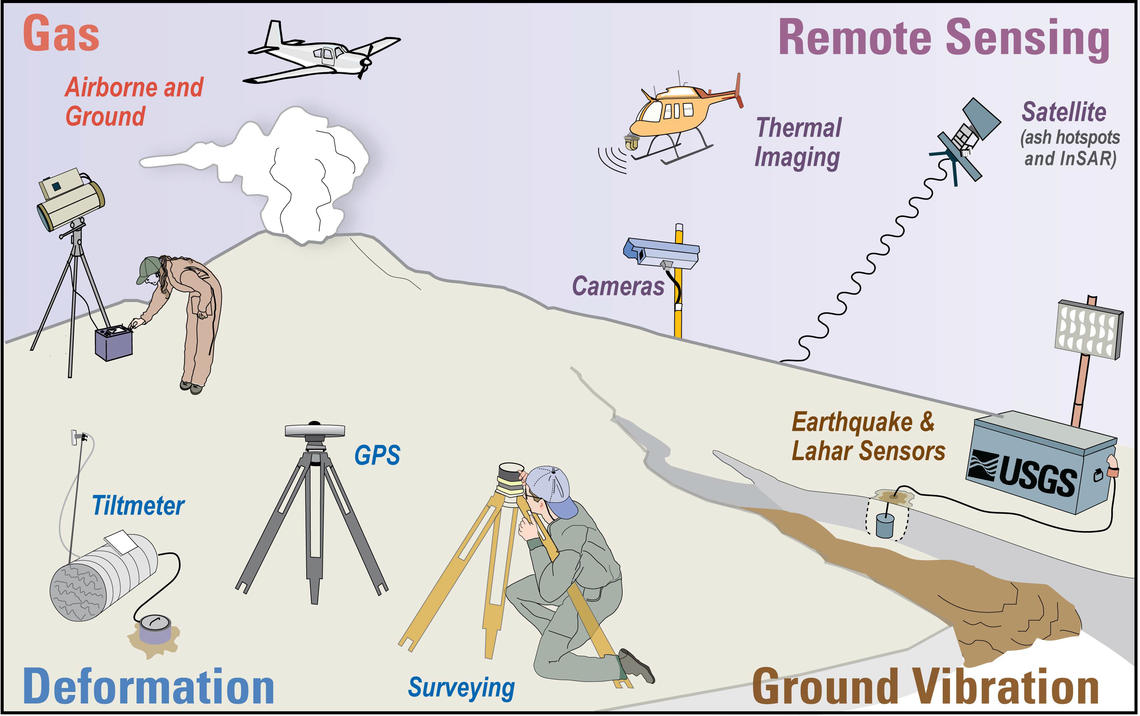
It is difficult to accurately predict whether or when a volcano will erupt, but scientists closely monitor a volcano over time to look for changes that could indicate geologic activity. Scientists will measure characteristics such as seismic activity surrounding the volcano, the deformation of the cone, gas emissions, and past history of volcanic eruptions [1,6]. These measurements will lead scientists to advise officials to take decisive action that can lead to evacuations, and hopefully, the prevention of a catastrophe.
History of Volcanic Activity
How active is a volcano? Scientists try to compile historical records from cities, towns, and villages surrounding a volcano to learn when, over the course of written history, it last erupted. Geologists can also examine the cooled lava fields along the slopes of a volcano, and even date some of the recently deposited ash and rock, to determine when it was last ejected from the vent.
Volcanoes are broadly placed into four categories: erupting, dormant, active, and extinct. An erupting volcano, as the name suggests, is currently having an ongoing eruption. A volcano that is not erupting, but still has a source of magma that enables it to eventually erupt one day is called dormant. If a volcano has erupted just once in the past 10,000 years, scientists consider it to be active, even if it is in a period of dormancy or currently erupting. The only “safe” volcano is classified as extinct, which means that it is not expected to erupt in the future. Nonetheless, even extinct volcanoes may surprise scientists and they still are prone to mass wasting events.
Scientists usually focus their monitoring efforts on active volcanoes, especially those that might affect populated areas. By documenting the eruption history of active volcanoes, scientists may even be able to construct a broad timeframe in which an eruption is expected.
Earthquakes
When magma stirs beneath an active volcano, it can shake the ground nearby. The sudden shaking of ground beneath a volcano produces energy in the form of seismic waves, or earthquakes.
A good indicator of a volcano that is just about to erupt is a series or “swarm” of earthquakes. Scientists measure these with instruments called seismographs, which capture the seismic waves released by the movement of the volcanic slope [7].
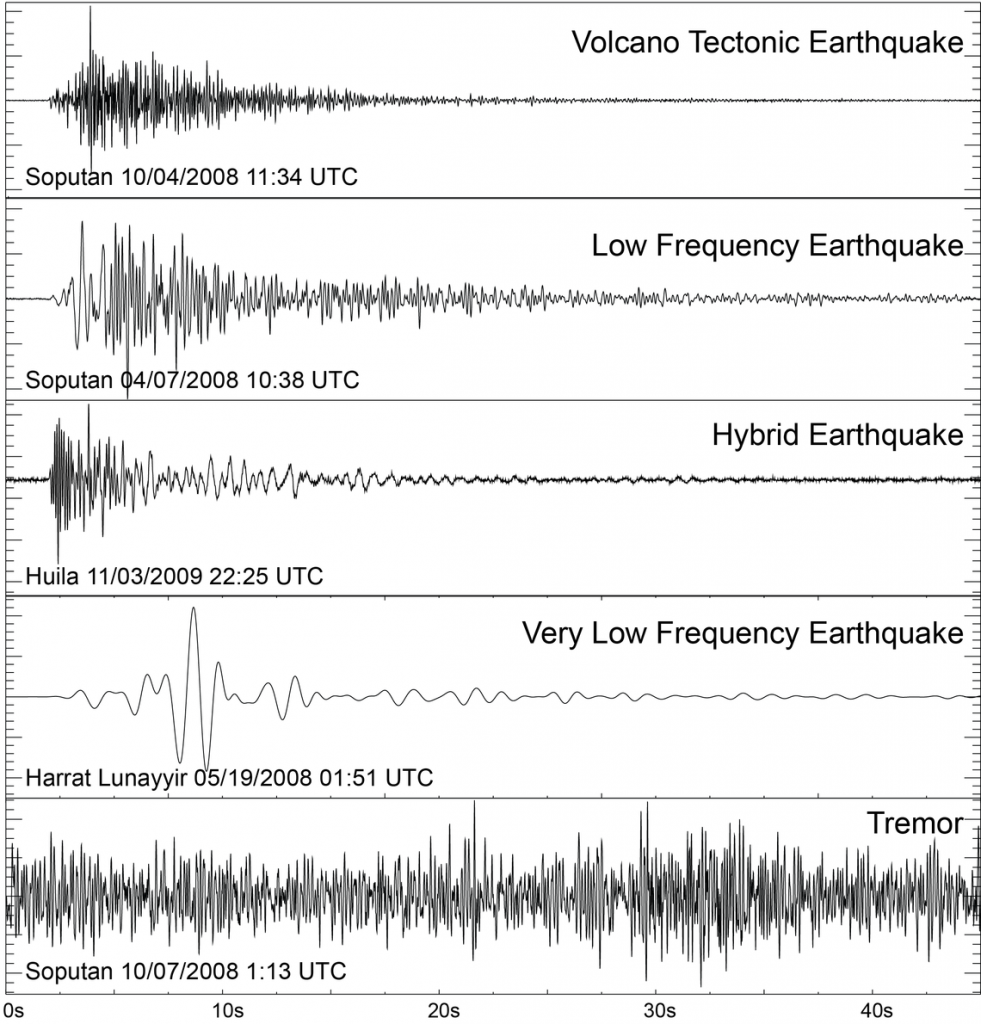
Ground Deformation
In addition to producing earthquakes, the movement of magma under a volcano can also push and move the flanks of the mountainside. The deformation of the ground along the volcanic slope is not very apparent to the naked eye. Scientists use instruments called tiltmeters, which precisely measure the angle of a volcano’s slope. When that slope starts to deform under pressure from the underlying melted rock and gas, it will change the slope angle of the volcano [8]. This process will even cause mass wasting events.
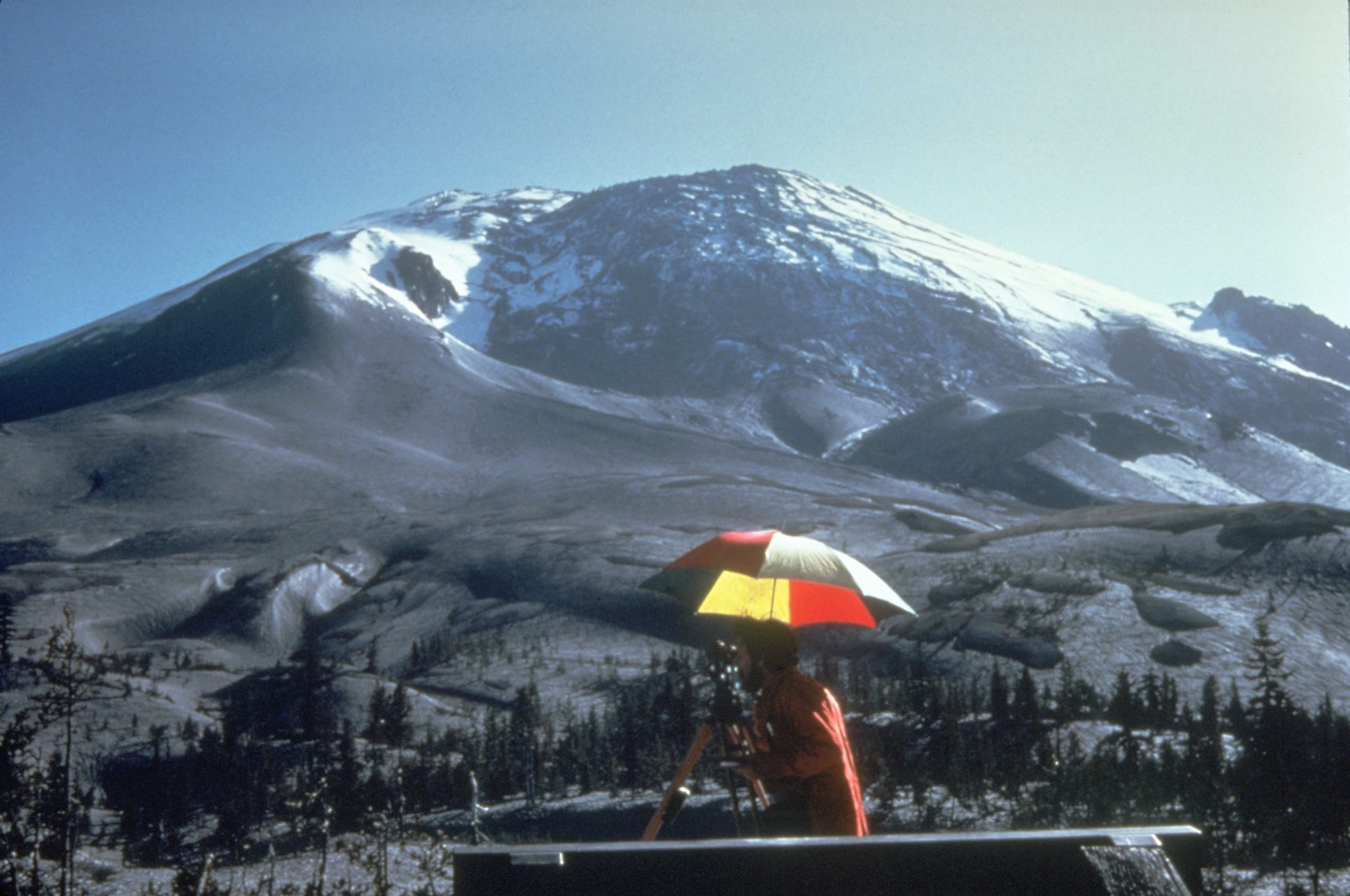
Some scientists now use the modern technique of remote sensing to detect subtle changes in the volcano’s shape and slope. Modern drones and satellites can be implemented to track small changes in elevation, temperature in areas that are inaccessible to scientists. Some of these technologies include Light Detection and Ranging (Lidar), Global Positioning System stations (GPS), and Interferometric Synthetic Aperture Radar (InSAR).
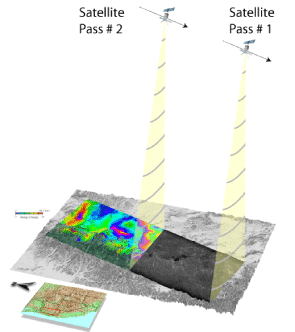
Gas Emissions
Some active volcanoes that are about to erupt release gases ahead of magma. These gases include sulfur dioxide (SO2), carbon dioxide (CO2), water vapor (H2O), and hydrochloric acid (HCl). Scientists will detect these gases near the vent of the volcano or sample them and analyze them later with sophisticated instruments that can measure gas concentration called spectrometers. If certain gases increase in concentration, it may indicate that an eruption is imminent [9].
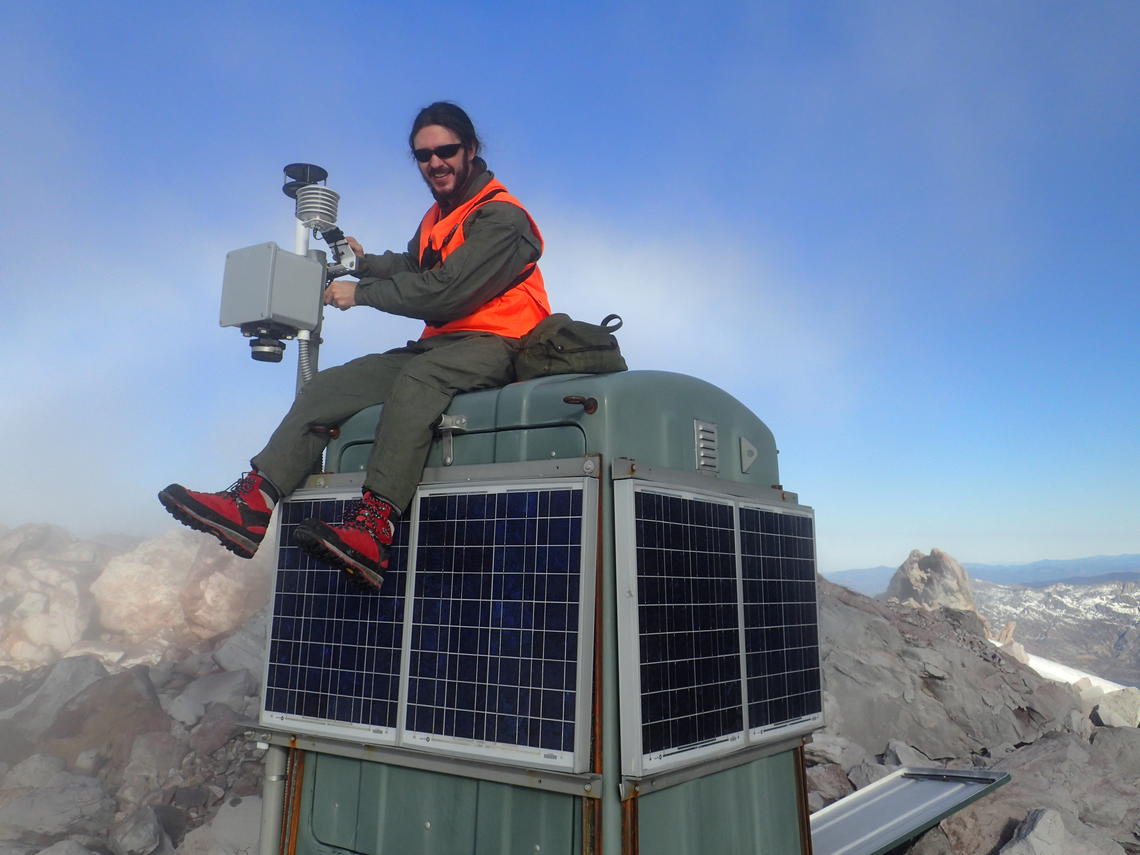
***See 5.8 for Text and Media Attributions
As previously discussed in section 3.9, a rock is a collection of consolidated minerals. Rocks are grouped into three main categories based on how they form:
- Igneous: formed from the cooling and crystallization of molten rock.
- Sedimentary: formed when weathered fragments of other rocks are buried, compressed, and cemented together, or when minerals precipitate directly from solution
- Metamorphic: formed by alteration (due to heat, pressure, and/or chemical action) of a pre-existing igneous or sedimentary rock
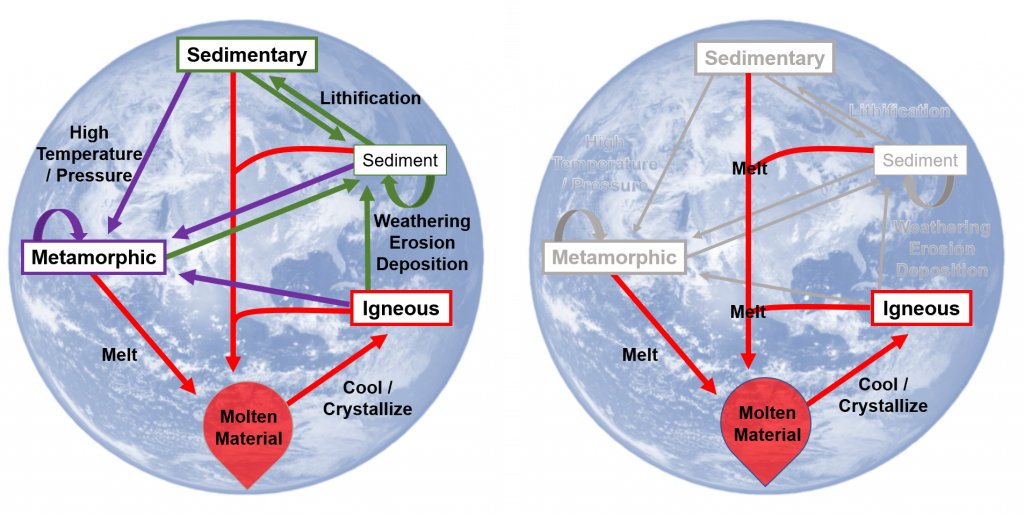
The focus of this chapter is on Igneous rocks, though it is important to remember this is just a piece of the rock cycle.
Learning Objectives
After carefully reading this chapter, completing the exercises within it, and answering the questions at the end, you should be able to:
- Describe the rock cycle and the types of processes that lead to the formation of igneous, sedimentary, and metamorphic rocks, and explain why there is an active rock cycle on Earth.
- Explain the concept of partial melting and describe the geological processes that lead to melting.
- Describe, in general terms, the range of chemical compositions of magmas.
- Discuss the processes that take place during the cooling and crystallization of magma, and the typical order of crystallization according to the Bowen reaction series.
- Explain how magma composition can be changed by fractional crystallization and partial melting of the surrounding rocks.
- Apply the criteria for igneous rock classification based on mineral proportions.
- Describe the origins of phaneritic, porphyritic, and pegmatitic rock textures.
- Identify plutons on the basis of their morphology and their relationships to the surrounding rocks.
- Explain the origin of a chilled margin.
an area on the Earth's surface where lava, ash, and/or volatile gases erupt and eventually solidify into rock.
As has already been described, igneous rocks are classified into four categories: felsic, intermediate, mafic, and ultramafic, based on either their chemistry or their mineral composition. The diagram in Figure 4.4.1 can be used to help classify igneous rocks by their mineral composition. An important feature to note on this diagram is the red line separating the non-ferromagnesian silicates in the lower left (K-feldspar, quartz, and plagioclase feldspar) from the ferromagnesian silicates in the upper right (biotite, amphibole, pyroxene, and olivine). In classifying intrusive igneous rocks, the first thing to consider is the percentage of ferromagnesian silicates. In most igneous rocks the ferromagnesian silicate minerals are clearly darker than the others, but it is still quite difficult to estimate the proportions of minerals in a rock.
Based on the position of the red line in Figure 4.4.1, it is evident that felsic rocks can have between 1% and 20% ferromagnesian silicates (the red line intersects the left side of the felsic zone 1% of the distance from the top of the diagram, and it intersects the right side of the felsic zone 20% of the distance from the top). Intermediate rocks have between 20% and 50% ferromagnesian silicates, and mafic rocks have 50% to 100% ferromagnesian silicates. To be more specific, felsic rocks typically have biotite and/or amphibole; intermediate rocks have amphibole and, in some cases, pyroxene; and mafic rocks have pyroxene and, in some cases, olivine.
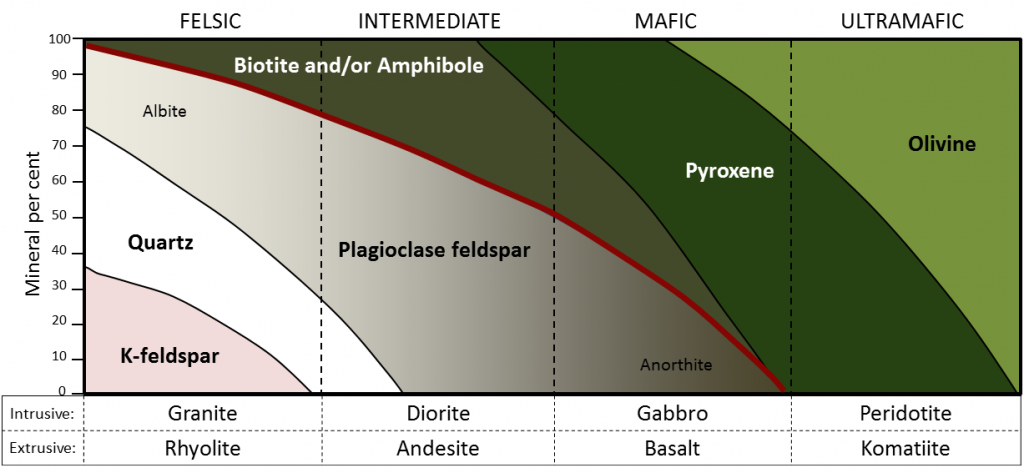
If we focus on the non-ferromagnesian silicates, it is evident that felsic rocks can have from 0% to 35% K-feldspar, from 25% to 35% quartz (the vertical thickness of the quartz field varies from 25% to 35%), and from 25% to 50% plagioclase (and that plagioclase will be sodium-rich, or albitic). Intermediate rocks can have up to 25% quartz and 50% to 75% plagioclase. Mafic rocks only have plagioclase (up to 50%), and that plagioclase will be calcium-rich, or anorthitic.
Exercise: Mineral proportions in igneous rocks

Figure 3.4.3 provides a diagrammatic representation of the proportions of dark minerals in light-coloured rocks. You can use that when trying to estimate the ferromagnesian mineral content of actual rocks, and you can get some practice doing that by completing Exercise 3.6. Be warned! Geology students almost universally over-estimate the proportion of dark minerals.

Exercise 3.6 Proportions of ferromagnesian silicates
The four igneous rocks shown below have differing proportions of ferromagnesian silicates.
Igneous rocks are also classified according to their textures. The textures of volcanic rocks will be discussed in Chapter 4, so here we’ll only look at the different textures of intrusive igneous rocks. Almost all intrusive igneous rocks have crystals that are large enough to see with the naked eye, and we use the term phaneritic (from the Greek word phaneros meaning visible) to describe that. Typically that means they are larger than about 0.5 millitmeres (mm) — the thickness of a strong line made with a ballpoint pen. (If the crystals are too small to distinguish, which is typical of most volcanic rocks, we use the term aphanitic (from the Greek word aphanos - unseen) The intrusive rocks shown in Figure 3.3.5 are all phaneritic, as are those shown in Exercise 3.6.
In general, the size of crystals is proportional to the rate of cooling. The longer it takes for a body of magma to cool, the larger the crystals can grow. It is not uncommon to see an intrusive igneous rock with crystals up to 1 centimetre (cm) long. In some situations, especially toward the end of the cooling stage, the magma can become water rich. The presence of liquid water (still liquid at high temperatures because it is under pressure) promotes the relatively easy movement of ions, and this allows crystals to grow large, sometimes to several centimetres (Figure 3.4.4). Finally, as already described, if an igneous rock goes through a two-stage cooling process, its texture will be porphyritic (Figure 3.3.7).

Image Descriptions
Attributions
- Figure 3.4.1, 3.4.2, 3.4.3: © Steven Earle. CC BY.
- Figure 3.4.4: Pegmatite. Public domain.
As has already been described, igneous rocks are classified into four categories: felsic, intermediate, mafic, and ultramafic, based on either their chemistry or their mineral composition. The diagram in Figure 4.4.1 can be used to help classify igneous rocks by their mineral composition. An important feature to note on this diagram is the red line separating the non-ferromagnesian silicates in the lower left (K-feldspar, quartz, and plagioclase feldspar) from the ferromagnesian silicates in the upper right (biotite, amphibole, pyroxene, and olivine). In classifying intrusive igneous rocks, the first thing to consider is the percentage of ferromagnesian silicates. In most igneous rocks the ferromagnesian silicate minerals are clearly darker than the others, but it is still quite difficult to estimate the proportions of minerals in a rock.
Based on the position of the red line in Figure 4.4.1, it is evident that felsic rocks can have between 1% and 20% ferromagnesian silicates (the red line intersects the left side of the felsic zone 1% of the distance from the top of the diagram, and it intersects the right side of the felsic zone 20% of the distance from the top). Intermediate rocks have between 20% and 50% ferromagnesian silicates, and mafic rocks have 50% to 100% ferromagnesian silicates. To be more specific, felsic rocks typically have biotite and/or amphibole; intermediate rocks have amphibole and, in some cases, pyroxene; and mafic rocks have pyroxene and, in some cases, olivine.
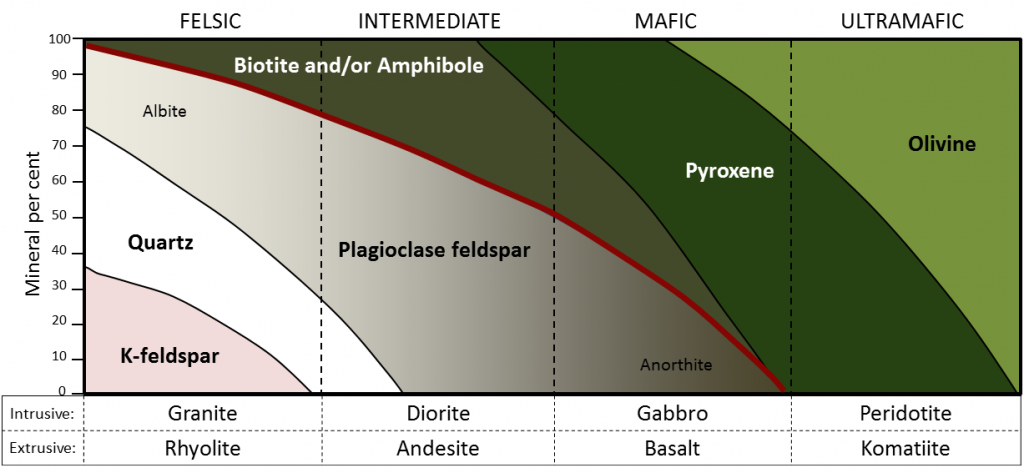
If we focus on the non-ferromagnesian silicates, it is evident that felsic rocks can have from 0% to 35% K-feldspar, from 25% to 35% quartz (the vertical thickness of the quartz field varies from 25% to 35%), and from 25% to 50% plagioclase (and that plagioclase will be sodium-rich, or albitic). Intermediate rocks can have up to 25% quartz and 50% to 75% plagioclase. Mafic rocks only have plagioclase (up to 50%), and that plagioclase will be calcium-rich, or anorthitic.
Exercise: Mineral proportions in igneous rocks

Figure 4.4.3 provides a diagrammatic representation of the proportions of dark minerals in light-colored rocks. You can use that when trying to estimate the ferromagnesian mineral content of actual rocks, and you can get some practice doing that by completing Exercise 3.6. Be warned! Geology students almost universally over-estimate the proportion of dark minerals.

Exercise: Proportions of ferromagnesian silicates
The four igneous rocks shown below have differing proportions of ferromagnesian silicates.
Igneous rocks are also classified according to their textures. The textures of volcanic rocks will be discussed in Chapter 4, so here we’ll only look at the different textures of intrusive igneous rocks. Almost all intrusive igneous rocks have crystals that are large enough to see with the naked eye, and we use the term phaneritic (from the Greek word phaneros meaning visible) to describe that. Typically that means they are larger than about 0.5 millimeters (mm) — the thickness of a strong line made with a ballpoint pen. (If the crystals are too small to distinguish, which is typical of most volcanic rocks, we use the term aphanitic (from the Greek word aphanos - unseen) The intrusive rocks shown in Figure 3.3.5 are all phaneritic, as are those shown in Exercise 3.6.
In general, the size of crystals is proportional to the rate of cooling. The longer it takes for a body of magma to cool, the larger the crystals can grow. It is not uncommon to see an intrusive igneous rock with crystals up to 1 centimeter (cm) long. In some situations, especially toward the end of the cooling stage, the magma can become water rich. The presence of liquid water (still liquid at high temperatures because it is under pressure) promotes the relatively easy movement of ions, and this allows crystals to grow large, sometimes to several centimeters (Figure 3.4.4). Finally, as already described, if an igneous rock goes through a two-stage cooling process, its texture will be porphyritic (Figure 4.3.7).

As has already been described, igneous rocks are classified into four categories: felsic, intermediate, mafic, and ultramafic, based on either their chemistry or their mineral composition. The diagram in Figure 4.4.1 can be used to help classify igneous rocks by their mineral composition. An important feature to note on this diagram is the red line separating the non-ferromagnesian silicates in the lower left (K-feldspar, quartz, and plagioclase feldspar) from the ferromagnesian silicates in the upper right (biotite, amphibole, pyroxene, and olivine). In classifying intrusive igneous rocks, the first thing to consider is the percentage of ferromagnesian silicates. In most igneous rocks the ferromagnesian silicate minerals are clearly darker than the others, but it is still quite difficult to estimate the proportions of minerals in a rock.
Based on the position of the red line in Figure 4.4.1, it is evident that felsic rocks can have between 1% and 20% ferromagnesian silicates (the red line intersects the left side of the felsic zone 1% of the distance from the top of the diagram, and it intersects the right side of the felsic zone 20% of the distance from the top). Intermediate rocks have between 20% and 50% ferromagnesian silicates, and mafic rocks have 50% to 100% ferromagnesian silicates. To be more specific, felsic rocks typically have biotite and/or amphibole; intermediate rocks have amphibole and, in some cases, pyroxene; and mafic rocks have pyroxene and, in some cases, olivine.
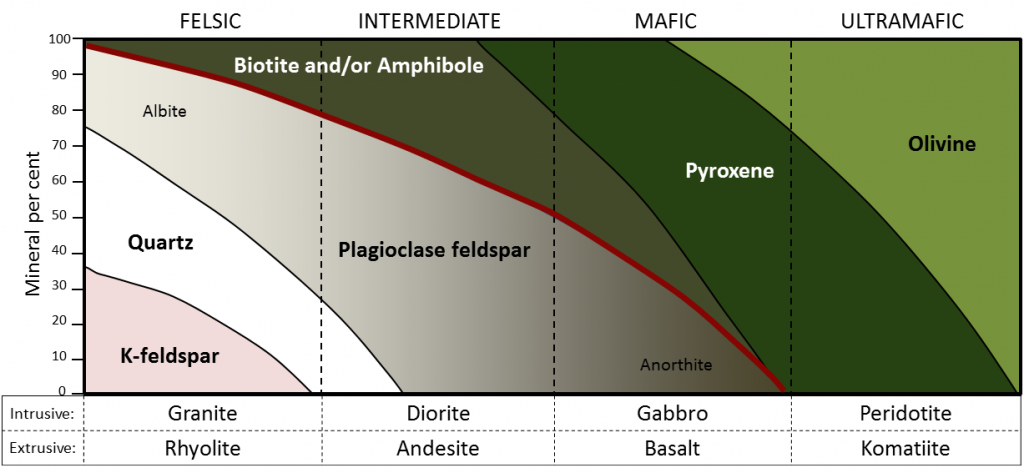
If we focus on the non-ferromagnesian silicates, it is evident that felsic rocks can have from 0% to 35% K-feldspar, from 25% to 35% quartz (the vertical thickness of the quartz field varies from 25% to 35%), and from 25% to 50% plagioclase (and that plagioclase will be sodium-rich, or albitic). Intermediate rocks can have up to 25% quartz and 50% to 75% plagioclase. Mafic rocks only have plagioclase (up to 50%), and that plagioclase will be calcium-rich, or anorthitic.
Exercise: Mineral proportions in igneous rocks

Figure 4.4.3 provides a diagrammatic representation of the proportions of dark minerals in light-colored rocks. You can use that when trying to estimate the ferromagnesian mineral content of actual rocks. Be warned! Geology students almost universally over-estimate the proportion of dark minerals.

Exercise: Proportions of ferromagnesian silicates
The four igneous rocks shown below have differing proportions of ferromagnesian silicates.
Igneous rocks are also classified according to their textures. The textures of volcanic rocks will be discussed in Chapter 4, so here we’ll only look at the different textures of intrusive igneous rocks. Almost all intrusive igneous rocks have crystals that are large enough to see with the naked eye, and we use the term phaneritic (from the Greek word phaneros meaning visible) to describe that. Typically that means they are larger than about 0.5 millimeters (mm) — the thickness of a strong line made with a ballpoint pen. (If the crystals are too small to distinguish, which is typical of most volcanic rocks, we use the term aphanitic (from the Greek word aphanos - unseen) The intrusive rocks shown in Figure 3.3.5 are all phaneritic, as are those shown in Exercise 3.6.
In general, the size of crystals is proportional to the rate of cooling. The longer it takes for a body of magma to cool, the larger the crystals can grow. It is not uncommon to see an intrusive igneous rock with crystals up to 1 centimeter (cm) long. In some situations, especially toward the end of the cooling stage, the magma can become water rich. The presence of liquid water (still liquid at high temperatures because it is under pressure) promotes the relatively easy movement of ions, and this allows crystals to grow large, sometimes to several centimeters (Figure 3.4.4). Finally, as already described, if an igneous rock goes through a two-stage cooling process, its texture will be porphyritic (Figure 4.3.7).

We may receive all the heat we need on the Earth's surface from sunlight, but if you dig a hole just a few feet deep, you might notice that the surrounding soil is much cooler. This happens because much of the sediment is insulated from the sun's energy. Nevertheless, if you had the ability to dig miles and miles into the crust, you would find that the temperature of the rock would start to significantly increase!

This increase in temperature with depth in the Earth's crust is called the geothermal gradient. In general, we can expect temperatures to increase about 25°C for each kilometer of depth. This sounds like a significant increase in heat, but once we pass the 100 km mark, temperatures really take off!
As we learned in the second chapter, the Earth's iron core is wickedly hot! Such heat powers plate tectonics, and when combined with other factors, it can lead to partial melting in the upper mantle. This melted, molten rock occasionally rises to the Earth's surface and when it does, watch out!
A volcano will form above a chamber or plume of magma. There is a wide variety of volcanoes, and some are more explosive than others. Molten lava is far from the only hazard; volcanic eruptions can cause massive damage with ashfall, pyroclastic flows, toxic gases, and deadly landslides. There are even a few volcanoes considered so dangerous that they are termed "supervolcanoes", and they have the potential to drastically impact society for generations.
Although volcanoes are hazardous, they are a continuous boon for the global economy. The precious minerals and useful rocks formed by volcanic processes have benefited civilization for thousands of years. In the modern economy, we use volcanism as a source of alternative energy and igneous rocks in trades ranging from construction to cosmetics.
Learning Objectives
- Describe the size, shape, and eruption style of volcanoes and how they form/
- Identify the major volcanic hazards and their effects on humans and property.
- Explain how scientists monitor volcanoes and where volcanoes can be found.
- Explain how volcanic processes benefit society.
Image by Karla Panchuk (CC BY 2.0)
molten rock that can be found beneath the Earth's surface.
Image by kuhnmi (Flickr) CC BY 2.0
Image by Steven Earle CC BY
Roy Luck (2009) CC BY 2.0; Pacaya aa: Greg Willis (2008) CC BY-SA 2.0