12 7.2 Classification of Metamorphic Rocks
There are two main types of metamorphic rocks: those that are foliated because they have formed in an environment with either directed pressure or shear stress, and those that are not foliated because they have formed in an environment without directed pressure or relatively near the surface with very little pressure at all. Some types of metamorphic rocks, such as quartzite and marble, which can form whether there is directed-pressure or not, do not typically exhibit foliation because their minerals (quartz and calcite respectively) do not tend to show alignment (see Figure 7.2.8).
Metamorphic Texture
Metamorphic texture describes the shape and orientation of mineral grains within a metamorphic rock. As the original rock is subjected to higher temperatures and pressures, some of its minerals might stretch out in a single direction, recrystallize, or enlarge. Therefore, the new metamorphic rock will have a different texture than the parent rock.
Metamorphic texture is broadly categorized as either Foliated or Non-Foliated.
Foliated Texture
Foliation is a term that describes how minerals line up along a preferred direction. Some minerals, particularly micas, are usually thin and planar by default. Rocks with foliated texture look like they have their minerals stacked together as though they were pages in a book; hence the term “folia”, or leaflike.
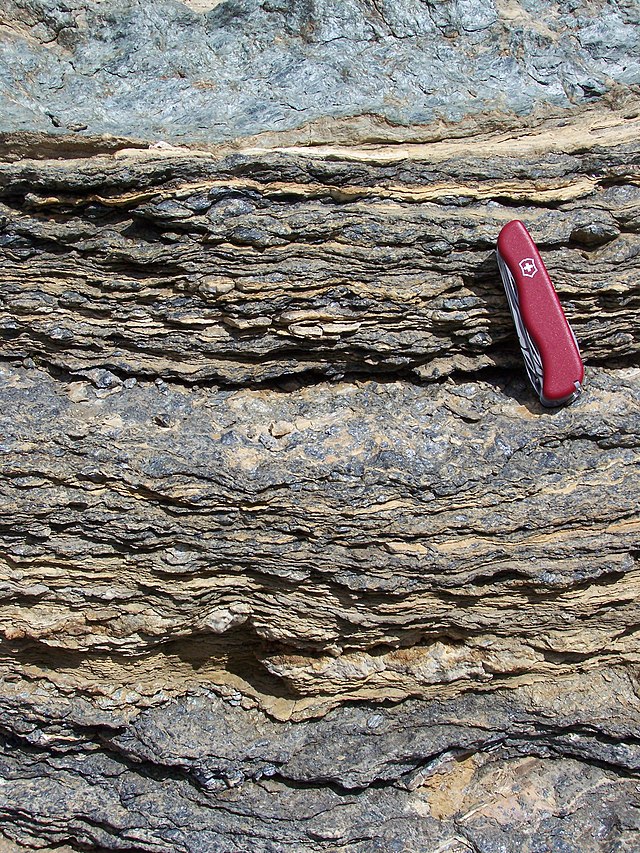
Why do foliated rocks form during metamorphism? At least two conditions need to be satisfied. First, the original rock must contain minerals that will easily deform or align with applied pressure into a flat plane. We know that mica minerals such as muscovite and biotite will do this as well as amphibole; however, stronger minerals like quartz and feldspar will often resist most pressure in the Earth’s crust.
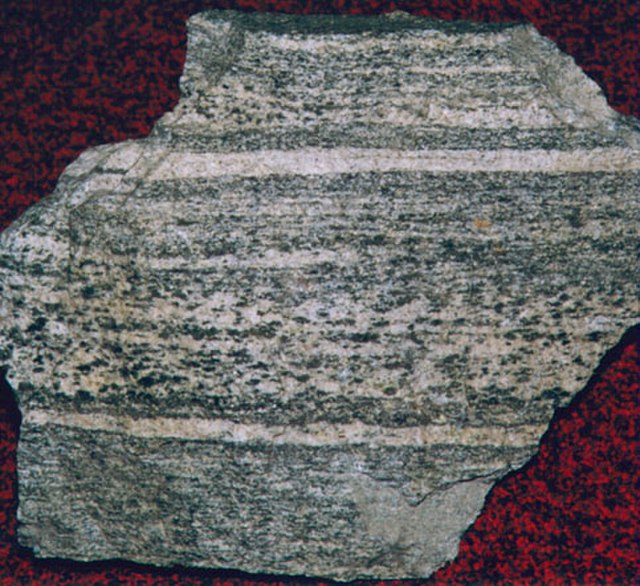
The second requirement needed for a foliated texture is directed pressure called differential stress. If pressure is applied unevenly, the weak minerals in the original rock will easily deform into long planes. This type of stress could be squeezing (compression), stretching (tensional), or sliding (shear) the rock unit.
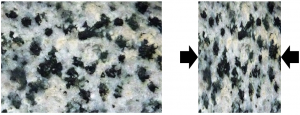
Non-Foliated Texture
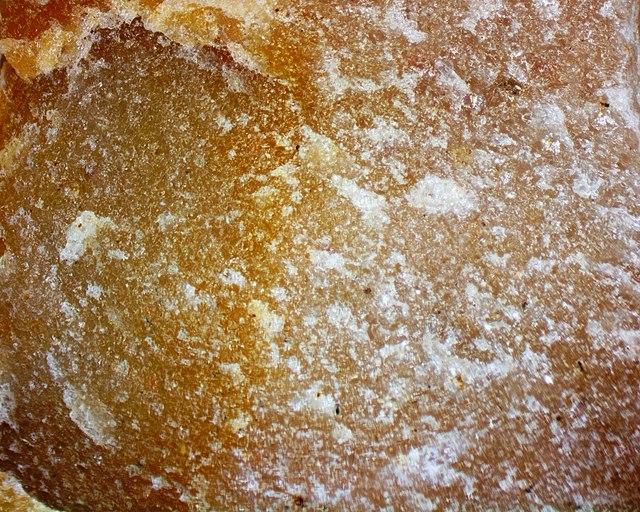
Non-foliated metamorphic rocks do not have any preferential alignments of mineral grains. These rocks are also called “Granoblastic“, which references the tendency of the individual grains to have somewhat equal shapes and dimensions. The majority of a non-foliated metamorphic rock contains mostly one mineral. Nonetheless, metamorphism has still taken place within these rocks; the mineral grains have recrystallized, interlocked, and grown larger. As a consequence, non-foliated rocks are much more durable and resistant to weathering than their parent rocks.
Metamorphic Grade
Metamorphic Grade refers to extent in which metamorphism can transform the the preexisting rock. This original rock is called the parent rock, and it can undergo low-grade metamorphism (little metamorphic change) to high-grade metamorphism (significant metamorphic change).
Low-grade metamorphism begins at temperatures and pressures that are not much higher than those that form sedimentary rocks. This type of metamorphism often results from rocks being buried at depths of at least 2 km. The conditions in such an environment are typically low-temperature and pressure. The parent rock still transforms into a new metamorphic rock; however, it is often easy to identify visual similarities between the resulting metamorphic rock and original rock.
High-grade metamorphism requires both high temperatures and pressures. Burial depths for high-grade metamorphic rocks can be up to 35 km! Mountain-building centers and subduction zones are prime examples of regions where high-grade metamorphism might take place. When a parent rock undergoes metamorphism at these conditions, the resulting metamorphic rock bears very few similarities to the original specimen; it often has strong foliation or banding of minerals.
Metamorphic rocks are often not restricted to either low or high-grade. Geologists typically rank these rocks on a scale of how many (or few) similarities they bear to the parent rock. In a sequence or outcrop filled with metamorphic rocks, you might observe some units that appear similar to the parent rock and others that look nothing like it. In general, the following rocks have been ranked in order of increasing metamorphic grade:
If a rock is buried to a great depth and encounters temperatures that are close to its melting point, it may partially melt. The resulting rock, which includes both metamorphosed and igneous material, is known as migmatite (Figure 7.2.5).
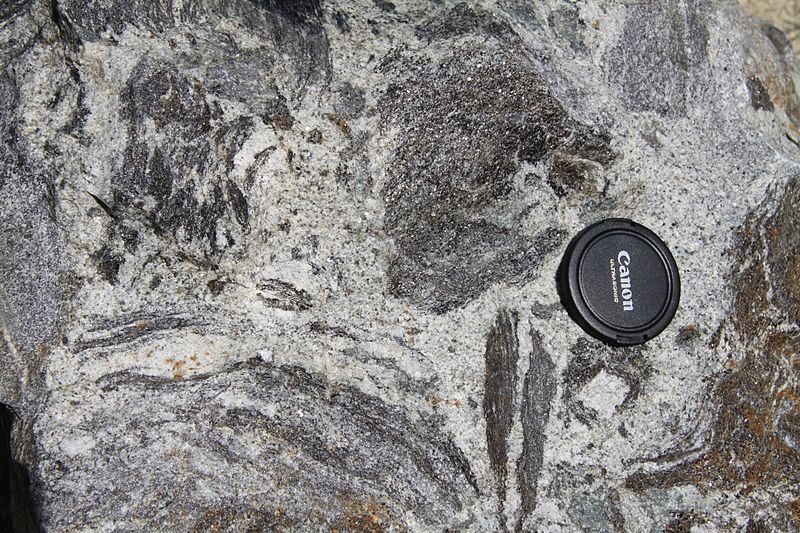
Parent Rocks
As already noted, the nature of the parent rock controls the types of metamorphic rocks that can form from it under differing metamorphic conditions. The kinds of rocks that can be expected to form at different metamorphic grades from various parent rocks are listed in Table 7.2. Some rocks, such as granite, do not change much at the lower metamorphic grades because their minerals are still stable up to several hundred degrees.
Parent Rock | Very Low Grade (150-300°C) | Low Grade (300-450°C) | Medium Grade (450-550°C) | High Grade (Above 550°C) |
---|---|---|---|---|
Mudrock | slate | phyllite | schist | gneiss |
Granite | no change | no change | almost no change | granite gneiss |
Basalt | chlorite schist | chlorite schist | amphibolite | amphibolite |
Sandstone | no change | little change | quartzite | quartzite |
Limestone | little change | marble | marble | marble |
A type of metamorphic texture in which the rock has repetitive layering or minerals lined along a preferred direction due to the uneven application of heat and pressure.
A process (usually in metamorphism) in which high temperatures and pressures cause the atoms within solid mineral structures to rearrange.
An original rock from which a new rock (usually metamorphic) is formed.
A type of metamorphic rock texture that indicates the lack of layering or preferred alignment of minerals. Non-foliated rocks are granular and usually contain interlocking mineral grains. Also known as granoblastic.
The arrangement of thin layers or repetitive structures in metamorphic rocks that results from the uneven applications of heat and pressure within the Earth's surface.
A group of silicate minerals called the "sheet" silicates. Mica minerals have their atomic structure arranged to easily pull apart as sheets in one direction.
The alteration of preexisting rock by elevated temperatures or pressures, usually beneath the Earth's surface.
A common mineral in the mica group that is light grayish-brown. It is flaky and peels apart in sheets.
A type of mica that is black or dark-colored and is typically found in igneous and metamorphic rocks.
a class of silicate minerals that are usually dark and form long crystals (as prisms or needles). Amphiboles are often magnesium (Mg) and iron (Fe) rich in composition.
A very common rock-forming silicate mineral with formula SiO2.
A group of silicate minerals which represents the most abundant mineral class of the continental crust.
The thin, outermost layer of Earth composed of rigid rock, which is home to all known life on the planet.
An uneven application of force against a material, such as a unit of rock, usually during metamorphism.
A type of force placed on an area of rock that presses or squeezes it tighter together.
A type of force placed on an area of rock that pulls or moves it apart.
A type of force placed on an area of rock that causes deformation with a sliding motion.
A non-foliated rock texture in metamorphic rocks, in which there is no preferred alignment of minerals or layering. Individual mineral grains of somewhat equal to one another in dimension.
rocks that cement together from weathering products, either from sediments or chemical ions in water.
The process in which the older, denser tectonic plate at a convergent boundary will buckle and sink into the lithosphere. This plate will always be composed of oceanic lithosphere.
Divergent boundaries are places where two or more plates move away from each other. These boundaries can be found on continental or oceanic lithosphere. Tensional stress operates between the tectonic plates at a divergent boundary, which causes the lithosphere at these locations to stretch and pull apart. Divergent boundaries slowly grow ocean basins along continental lithosphere.
The process of divergence is responsible for breaking up continental landmasses and supercontinents. This boundary type is the reason why the generations of scientists noticed the apparent fit between the coastlines of South America and Africa. Indeed, it is the reason that the world map appears the way it does today!
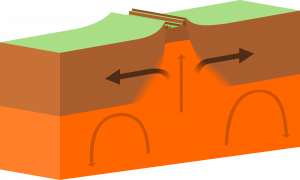
Continental Rift Valleys
How does a continent, or even a supercontinent, break apart? At first glance, such a task seems difficult considering continental lithosphere can be up to 20 times thicker than oceanic lithosphere due to billions of years of mountain-building. However, the insulation from that thick lithosphere will eventually allow a hot plume of magma from the mantle to rise toward the Earth's surface. That mantle plume will weaken the continental lithosphere, and eventually the convection cells within the mantle will be able to push the continent apart.
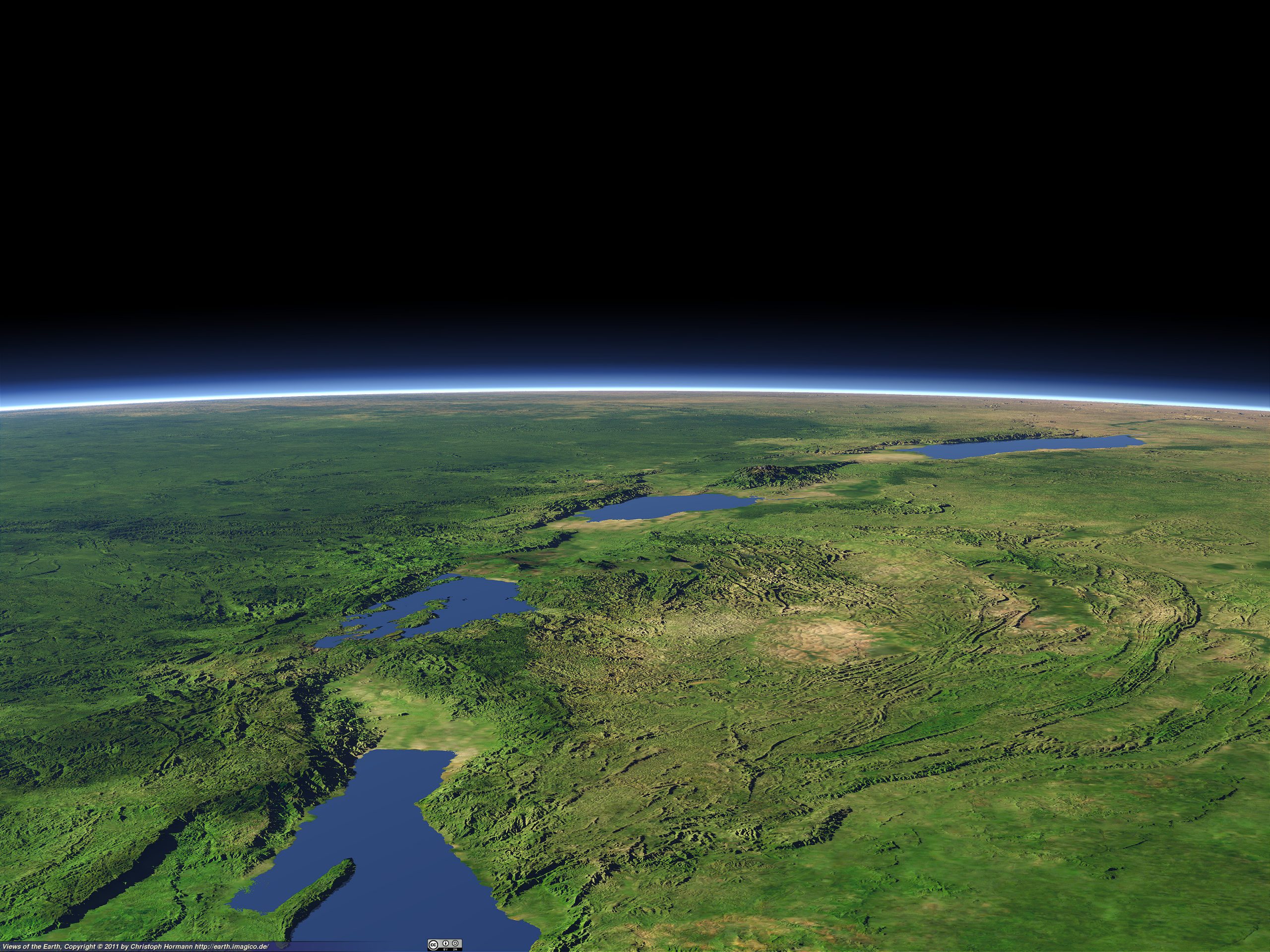
A continental rift valley is a region where the continental lithosphere is weakening and stretching apart. It is an observable divergent boundary and a sign that a continent is breaking apart. Given enough time, the rifting will continue to a point in which the continental lithosphere will become so thin, it will become predominantly enriched in the mantle plume materials. At that point, the rift will have created brand new oceanic crust, and a newborn ocean basin will open between the continental fragments.
This process of continental rifting has taken place about 250 million years ago between South America and Africa, as well as North America and Eurasia during the breakup of the supercontinent Pangaea. From a single landmass, the Earth saw many new continents form as well as a narrow sea that would one day widen to become our Atlantic Ocean.
https://www.youtube.com/watch?v=pf5KPXP4exc
PLATE TECTONICS IN ACTION: CONTINENTAL RIFT VALLEYS
Some of the world's continents are breaking apart as we speak! Here are some examples of regions undergoing continental rifting:
- The East African Rift Valley (Djibouti, Ethiopia, Uganda, Kenya, Tanzania)
- The Red Sea (Egypt, Sudan, Eritrea, Djibouti, Saudi Arabia, Yemen)
- Lake Baikal (Russia)
Mid-Ocean Ridges
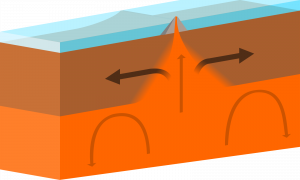
We have previously discussed the discovery of Mid-Ocean Ridges as a key piece of evidence for the theory of plate tectonics. The reason these spreading centers along the ocean floor are essential for plate tectonics is that they represent a clear example divergent boundary.
Mid-Ocean Ridges typically follow the long process of continental rifting. Once continental lithosphere has thinned and become mafic, upwelling magma begins to produce new oceanic lithosphere and a narrow ocean basin forms between the rifted continents (review video in previous section). As this process evolves, a Mid-Ocean Ridge forms above the rift on the floor of the new ocean because the cooling lava is hot and less dense (i.e., it takes up more space than cold material).
The places where we find Mid-Ocean Ridges are called spreading centers. These are sometimes characterized as "conveyer-belts" because they are the one site on the planet where new oceanic lithosphere is constantly created. These spreading centers are volcanoes; molten magma from the asthenosphere erupts underwater as lava and rapidly cools as new oceanic lithosphere. The material near the spreading centers is relatively hot and less dense, and therefore it has a higher elevation. Mid-Ocean Ridges often form long, high-elevation mountain chains because of their underwater volcanism.
Some Like It Boiling: Life at Hydrothermal Vents
Mid-ocean ridges also are home to some of the unique ecosystems discovered, found around hydrothermal vents that circulate ocean water through the shallow oceanic crust, and send it back out to rich chemical compounds and heat. While it was known for some time that hot fluids could be found on the ocean floor, it was only in 1977 when a team of scientists using the Diving Support Vehicle Alvin discovered a thriving community of organisms, including tubeworms bigger than people!
https://www.youtube.com/watch?v=R1koFEKfmLw
This group of organisms is dependent on the sun and photosynthesis but instead relies on chemical reactions with sulfur compounds and heat from within the Earth, a process known as chemosynthesis. Before this discovery, the thought in biology was that the sun was the ultimate source of energy in ecosystems; now, we know this to be false. Not only that, but some have also suggested it is from this that life could have started on Earth, and it now has become a target for extraterrestrial life (e.g., Jupiter's moon Europa).
Continued volcanism will cause the new oceanic lithosphere to move away from the Mid-Ocean Ridges, and that lithosphere will cool and sink deeper along the ocean floor. Because new oceanic lithosphere is always produced at a spreading center, the age of the seafloor is predictably younger near a Mid-Ocean Ridge and older as it moves farther away.
Divergent plate motion at a Mid-Ocean Ridge occurs at different rates, depending on the location. Spreading can be as fast as 20 cm/year or as slow as 1 cm/year. At these rates over hundreds of millions of years, it is not a surprise that the continents have moved so far apart.
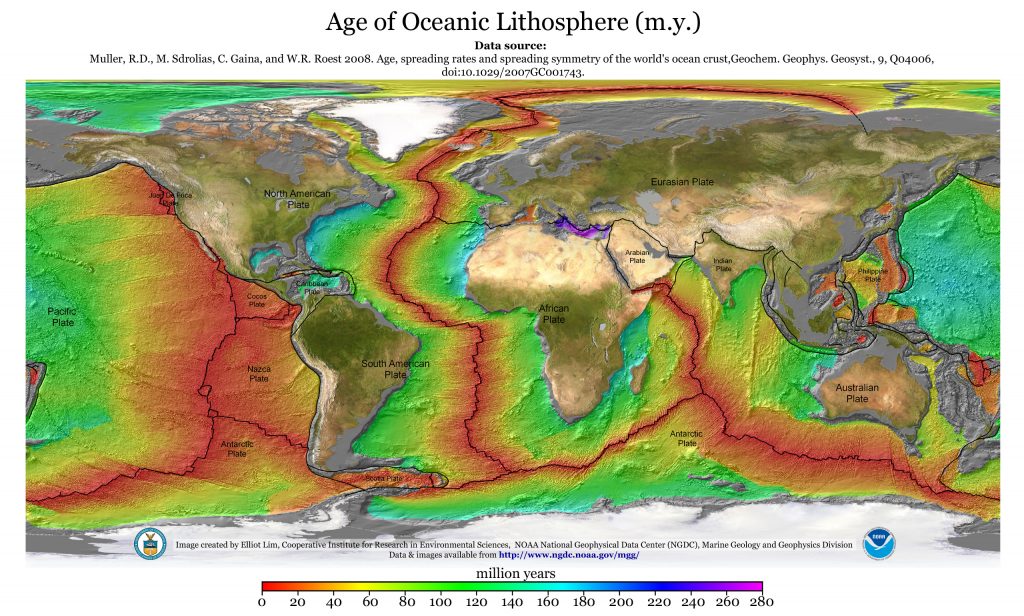
PLATE TECTONICS IN ACTION: MID-OCEAN RIDGES
Although they are sometimes deep underwater, we have known about mid-ocean ridges for decades! Here are some good examples of this type of divergent boundary:
- The Mid-Atlantic Ridge
- The East Pacific Rise
- The Gakkel Ridge/ Mid-Arctic Ridge